新闻动态
...of Nonimmunologic Loss of Transplanted Islets in Monkeys...
2025-06-13
American Journal of TransplantationVolume 14, Issue 7 p. 1543-1551 Original Article Free Access Prevention of Nonimmunologic Loss of Transplanted Islets in Monkeys M. Koulmanda, Corresponding Author Departments of Medicine and Surgery, The Transplant Institute, Beth Israel Deaconess Medical Center/Harvard Medical School, Boston, MACorresponding authors: Terry B. Strom, tstrom@bidmc.harvard.edu and Maria Koulmanda, mkoulman@bidmc.harvard.eduSearch for more papers by this authorR. S. Sampathkumar, Departments of Medicine and Surgery, The Transplant Institute, Beth Israel Deaconess Medical Center/Harvard Medical School, Boston, MASearch for more papers by this authorM. Bhasin, Genomics and Proteomics Core Center, Beth Israel Deaconess Medical Center/Harvard Medical School, Boston, MASearch for more papers by this authorA. Qipo, Departments of Medicine and Surgery, The Transplant Institute, Beth Israel Deaconess Medical Center/Harvard Medical School, Boston, MASearch for more papers by this authorZ. Fan, Departments of Medicine and Surgery, The Transplant Institute, Beth Israel Deaconess Medical Center/Harvard Medical School, Boston, MASearch for more papers by this authorG. Singh, Departments of Medicine and Surgery, The Transplant Institute, Beth Israel Deaconess Medical Center/Harvard Medical School, Boston, MASearch for more papers by this authorB. Movahedi, Departments of Medicine and Surgery, The Transplant Institute, Beth Israel Deaconess Medical Center/Harvard Medical School, Boston, MASearch for more papers by this authorM. Duggan, Department of Surgery, Center for Comparative Medicine, Massachusetts General Hospital, Boston, MASearch for more papers by this authorV. Chipashvili, Departments of Medicine and Surgery, The Transplant Institute, Beth Israel Deaconess Medical Center/Harvard Medical School, Boston, MASearch for more papers by this authorT. B. Strom, Corresponding Author Departments of Medicine and Surgery, The Transplant Institute, Beth Israel Deaconess Medical Center/Harvard Medical School, Boston, MACorresponding authors: Terry B. Strom, tstrom@bidmc.harvard.edu and Maria Koulmanda, mkoulman@bidmc.harvard.eduSearch for more papers by this author M. Koulmanda, Corresponding Author Departments of Medicine and Surgery, The Transplant Institute, Beth Israel Deaconess Medical Center/Harvard Medical School, Boston, MACorresponding authors: Terry B. Strom, tstrom@bidmc.harvard.edu and Maria Koulmanda, mkoulman@bidmc.harvard.eduSearch for more papers by this authorR. S. Sampathkumar, Departments of Medicine and Surgery, The Transplant Institute, Beth Israel Deaconess Medical Center/Harvard Medical School, Boston, MASearch for more papers by this authorM. Bhasin, Genomics and Proteomics Core Center, Beth Israel Deaconess Medical Center/Harvard Medical School, Boston, MASearch for more papers by this authorA. Qipo, Departments of Medicine and Surgery, The Transplant Institute, Beth Israel Deaconess Medical Center/Harvard Medical School, Boston, MASearch for more papers by this authorZ. Fan, Departments of Medicine and Surgery, The Transplant Institute, Beth Israel Deaconess Medical Center/Harvard Medical School, Boston, MASearch for more papers by this authorG. Singh, Departments of Medicine and Surgery, The Transplant Institute, Beth Israel Deaconess Medical Center/Harvard Medical School, Boston, MASearch for more papers by this authorB. Movahedi, Departments of Medicine and Surgery, The Transplant Institute, Beth Israel Deaconess Medical Center/Harvard Medical School, Boston, MASearch for more papers by this authorM. Duggan, Department of Surgery, Center for Comparative Medicine, Massachusetts General Hospital, Boston, MASearch for more papers by this authorV. Chipashvili, Departments of Medicine and Surgery, The Transplant Institute, Beth Israel Deaconess Medical Center/Harvard Medical School, Boston, MASearch for more papers by this authorT. B. Strom, Corresponding Author Departments of Medicine and Surgery, The Transplant Institute, Beth Israel Deaconess Medical Center/Harvard Medical School, Boston, MACorresponding authors: Terry B. Strom, tstrom@bidmc.harvard.edu and Maria Koulmanda, mkoulman@bidmc.harvard.eduSearch for more papers by this author Give accessShare full text accessShare full-text accessPlease review our Terms and Conditions of Use and check box below to share full-text version of article.I have read and accept the Wiley Online Library Terms and Conditions of UseShareable LinkUse the link below to share a full-text version of this article with your friends and colleagues. Learn more.Copy URLShare a linkShare onEmailFacebookTwitterLinked InRedditWechat Abstract The nonimmunologic loss of islets in the pre-, peri-, and early post-islet transplant periods is profound. To determine the potential role that transplantation of only a marginal mass of functioning beta cells may play in triggering late nonimmunologic graft loss, we studied the effect of treatment with alpha-1-antitrypsin (AAT) in the autologous cynomolgus islet transplant model. A marginal mass of autologous islets, that is islets prepared from 70% to 80% of the pancreas, was transplanted at 1600–4100 IEQ/kg into subtotal pancreatectomized, streptozotocin-treated and insulin-deficient diabetic hosts. In this marginal mass islet transplant model, islet function is insidiously lost over time and diabetes recurs in all untreated monkeys by 180 days posttransplantation. Short-term treatment with AAT, an acute phase reactant, in the peri-transplant period serves to terminate inflammation through effects upon expression of TGFβ, NFκB and AKT and favorably altering expression of cell death and survival pathways, as detected by a system biology approach and histology. These effects enabled functional expansion of the islet mass in transplanted hosts such that graft function improves rather than deteriorating over time. Abbreviations AAT alpha-1-antitrypsin ANGPT angiopoietin CCL carcinoma cell line ERK extracellular-signal-regulated kinases FOXP forkhead box P GAPDH glyceraldehyde 3-phosphate dehydrogenase H&E hematoxylin and eosin IEQ islet equivalents i.m. intra-muscular i.v. intravenous IVGTT intravenous glucose tolerance test JNK NH2-terminal kinase LCB lower confidence bound MAPK mitogen-activated protein kinase NFκB nuclear factor kappa-light-chain-enhancer of activated B cells NOD nonobese diabetic NS normal saline PLIER Probe Logarithmic Intensity Error SGOT serum glutamic oxaloacetic transaminase STZ streptozotocin T1D type 1 diabetes THY thymine Wnt wingless-int Introduction Insulin therapy provides an imperfect solution for the long-term care of patients with type 1 diabetes (T1D). In theory, clinical application of islet transplantation, particularly with the creation of immune tolerance, offers great hope for eluding the complications of T1D or of whole-organ transplantation. Yet the clinical application of islet transplantation has been slowed by the inability to routinely utilize islets from a single donor to restore and maintain long-term euglycemia in recipients of allogeneic transplants 1. Clearly, many islets perish in the harvesting and purification process and many more are lost shortly following engraftment as a result of nonimmunologic processes 2, 3. Until this problem is solved whole-organ transplantation, an intrusive and complication-prone procedure, will remain as the treatment of choice because a single whole-pancreas transplant routinely provides an islet cell mass sufficient to control hyperglycemia in the recipient for lengthy periods of time. It is possible that the modest islet cell mass surviving the pre-, peri- and immediate post-islet transplant procedures renders the recipient prone to beta cell exhaustion. Islet cell exhaustion may contribute to the long-term failure rate of islet transplants 4. Alpha-1-antitrypsin (AAT) is an acute phase reactant with anti-inflammatory, anti-leukocyte migratory, anti-thrombotic and anti-apoptotic effects 5-9. As expression of AAT sharply rises in response to inflammation, it seems reasonable to speculate that the function of this protein is to limit the duration, magnitude and perhaps molecular texture of inflammation 10. Moreover, AAT is known to exert cytoprotective effects upon cultured islets 8, 11 and, more importantly, syngeneic mouse islet transplants in the immediate posttransplant period 12. For these reasons, we have now studied the effect of short-term, peri-transplant AAT treatment in the cynomolgus islet autograft model in which insidious loss of insulin production and diabetes is the universal outcome by 180 days posttransplantation 4. Seventeen cynomolgus monkeys (Macaca fascicularis) weighing between 3.0 kg and 6.8 kg were obtained from Alpha Genesis, Inc. (Yemassee, SC), and quarantined for 6 weeks at the Massachusetts General Hospital, an institution fully accredited by the American Association for the Accreditation of Laboratory Animal Care (AAALAC), before the study. These monkeys were sero-negative for herpes B-virus, SIV, STLV-1, SRV, TB and hemagram parasites. Care of the monkeys was in accordance with \"good laboratory practice” regulations for nonclinical laboratory studies. Monkeys were assigned to the control and experimental groups by matching pairs for age and weight throughout the study. After overnight fasting, anesthesia was induced with ketamine 10–15 mg/kg intramuscular (i.m.) and maintained with inhalation of 1–2% isoflurane. Monkeys were hydrated with 50–60 mL of normal saline (NS). Partial pancreatectomy (70–80%) was performed, and the excised pancreas was processed for islet isolation. The remnant islet cell mass in the partially pancreatectomized host was destroyed through administration of streptozotocin (STZ; Sigma, St. Louis, MO). STZ was diluted in 10 mL of NS. Immediately after dilution, STZ was given at a dose of 55 mg/kg by rapid intravenous (i.v.) injection 3. Additional i.v. hydration with 100–150 mL of NS was given just following the infusion of STZ. Whole blood glucose levels were tested three times daily using an Accu–Check blood glucose monitor (Roche, Indianapolis, IN). As previously described 4, each partially pancreatectomized monkey used in this islet transplant model and then treated with rapid infusion of freshly prepared and sunlight-protected STZ, this is key, became permanently diabetic and insulin deficient. In this study, the diabetic monkeys were treated with two to three subcutaneous insulin injections per day (6–15 units a day) and given NS 100 mL/i.v. twice a week both pre- and post-transplantation for blood glucose levels over 200 mg/dL. The following tests were performed once or twice weekly posttransplantation: a complete blood count, electrolytes, serum creatinine, blood urea nitrogen, SGOT, alkaline phosphatase and bilirubin. Serum c-peptide levels were measured by radioimmunoassay (Human c-peptide RIA Kit; Linco Research, Inc., St. Charles, MO) two to three times a week. The assay for human c-peptide has 90% cross-reactivity with cynomolgus monkey c-peptide. Autologous islets were isolated on the day of transplantation from the excised pancreases 13. Pancreases with a warm ischemia time of less than 3 min were perfused via the pancreatic duct with University of Wisconsin solution and then transported on ice to the Joslin Diabetes Center or Beth Israel Deaconess Medical Center islet isolation core facility. The cold ischemia time was always less than 1 h. The pancreas was distended with an intra-ductal injection of Liberase HI (Roche Biochemicals, Indianapolis, IN) and incubated at 37°C in a static digestion chamber for 45 min. The digested tissue was collected and applied to a three-layer discontinuous Euroficoll gradient 13. Following purification, three 50 μL samples were stained with dithizone and counted to assess total islet cell yield 13. Islet samples were also taken for assessment of purity and viability on the day of isolation and 24 h later (time of transplantation). DNA, insulin content and insulin staining were not performed on all pancreases due to the small number of islets in some of the isolations. Finally, the total number of pancreatic islets obtained from the harvest and purification procedures was calculated as islet equivalents (IEQ) with an average diameter of 150 µm per islet. The islets were then cultured overnight. Table S1 summarizes the purity, viability and IEQ numbers of islets used for transplantation in all recipients. Diabetic recipients were anesthetized using ketamine 10–15 mg/kg/i.m. and anesthesia was maintained with ketamine 10–15 mg/kg i.v. and valium 1 mg/kg i.v. or i.m. during surgery. A mesenteric venous branch was cannulated with a 22-gauge catheter then passed into the portal circulation. In nine recipients, the islets were infused into portal circulation over a period of 2–3 min in a 10–20 mL volume of 0.9% NaCl 13. In eight additional recipients, the islets were transplanted beneath the renal capsule, a site convenient for graft harvest and thus transcriptional profiling analysis. The opening in the renal capsule was closed with 5–0 silk sutures 14. Following islet transplantation, blood glucose levels were checked twice daily. Insulin was not administered unless fasting blood glucose levels were above 150 mg/dL for two successive measurements. Graft failure was defined as the first day of 3 consecutive days of fasting blood glucose levels above 250 mg/dL unless the blood glucose levels subsequently normalized without insulin therapy. Food was withheld for 15 h before performing intravenous glucose tolerance tests (IVGTTs) monthly, and the monkeys were then given 0.5 g/kg glucose as a 25% glucose solution infused i.v. over 1 min. Blood samples were collected at 0, 1, 3, 5, 10, 15, 20, 25, 30, 45 and 60 min after infusion of the glucose solution and blood glucose levels were measured by glucometer. Aralast (human α-proteinase inhibitor; Baxter Healthcare Co., Westlake Village, CA) is an AAT product obtained from human blood. AAT is a major serum serine-protease inhibitor that inhibits the enzymatic activity of numerous serine-proteases including neutrophil elastase, cathespin G, proteinase 3, thrombin, trypsin and chymotrypsin 8. AAT was dosed at 60 mg/kg/i.v. at a rate of 0.33 mL/min or 19 drops per minute with a 60 drop/mL i.v. on transplant days −1, 3, 7 and 14. The transcriptional profile of islets placed beneath the renal capsule from control and AAT-treated primates was obtained by oligonucleotide microarray analysis using HT U133 Plus PM Array plates (Affymetrix, Santa Clara, CA) presenting over 54 000 probe sets, allowing for the analysis of 47 000 transcripts, representative of over 33 000 well-characterized human genes. Human gene chips are used for primate samples due to the high evolutionary conservation of primate to human gene sequences. The array analysis at the Beth Israel Deaconess Medical Center Genomics, Proteomics, Bioinformatics and Systems Biology Center was conducted according to the standard Affymetrix protocol using high-throughput hybridization and scanning system conducted transcriptional profiling. All experiments were performed at least in duplicate. The quality of hybridized chips was assessed using Affymetrix guidelines on the basis of PM mean, 3′ to 5′ ratios for beta-actin and GAPDH and values for spike-in control transcripts. Reproducibility of the samples was checked by using chip-to-chip correlation and signal-to-noise ratio methods for replicate arrays using \"arrayQualityMetrics,” a bio-conductor package 15. All the high-quality arrays were included for unsupervised and supervised bioinformatics analysis. To obtain signal values, chips were further analyzed using the Probe Logarithmic Intensity Error (PLIER) algorithm, a system more robust than Affymetrix software Microarray Analysis Suite 5.0 in signal calculation, as it uses a probe affinity parameter representing the strength of a signal produced at a specific concentration for a given probe. This algorithm was shown to produce improved signal values by accounting for experimental variation pattern as well as error at low and high gene intensity. The transcripts with low absolute intensity were filtered out from further analysis. When comparing islets from untreated (control) versus AAT-treated hosts, we used the lower confidence bound (LCB) of the fold change to identify differentially expressed genes from normalized data that was also shown (data not shown) as the best method for identifying differentially expressed genes form experiments with small sample sizes. If the 90% LCB of the fold change between islets from untreated and treated hosts was over 1.5, the corresponding gene is differentially expressed. Further, interactive networks, pathways and function analysis were performed on significantly differentially expressed genes using Ingenuity Pathways Analysis (IPA 7.0; www.ingenuity.com), a commercial system biology oriented package. The knowledge base of this software consists of functions, pathways and network models derived by systematically exploring the peer-reviewed scientific literature. It calculates p-values using Fisher\'s exact test for each pathway and functions according to the fit of user\'s data to IPA database. The p-value measures how likely the observed association between a specific pathway/function/interactive network and the data set would be if it were only due to random chance by also considering the total number of functions, pathways and interactive network eligible genes in your data set and the reference set of genes (those which potentially could be significant in the given data set). Focus molecules were identified from the integrated networks on the basis of degree of connectivity (number of interactions for each gene). The focus hubs with highest degrees of connectivity are considered critical for maintenance of the networks, suggesting that therapeutic targeting of these focus hubs may elicit the strongest impact. Differences between groups (AAT-treated islet autograft vs. untreated) were tested by the independent-samples t-test using SPSS 17 statistical software (IBM, Armonk, NY). A p-value 0.05 was considered to be significant. Control group monkeys rendered diabetic by partial pancreatectomy followed by administration of STZ received autologous islet transplants. In each of these eight recipients receiving islets in the portal vein or underneath the renal capsule, the blood glucose levels sharply decreased within 4 days of transplantation and both fasting and nonfasting blood glucose levels remained less than 150 mg/dL for ca. 80–90 days. Blood glucose levels then slowly deteriorated. In four of these monkeys, the islets were placed under the kidney capsule. Recipients bearing islets beneath the kidney capsule were sacrificed when blood glucose levels reached 180–200 mg/dL. This occurred around 100–120 days posttransplantation. At this time, the kidney bearing the transplant was harvested for transcriptional profiling studies. In the other four control monkeys, islet autografts were infused into the portal circulation in hosts also rendered diabetic by partial pancreatectomy followed by STZ infusion. As noted above in all such subjects, fasting blood glucose levels rose to 150 mg/dL ca. 80–90 days posttransplantation (Figure 1A) as c-peptide levels deteriorate (Figure 1B) leading to frank diabetes in control recipients within 150–180 days posttransplantation. Interestingly, the insidious loss of autograft function in untreated hosts was most rapid in grafts with the most obviously marginal initial graft function posttransplantation (data not shown). IVGTT data revealed deterioration as early as 90 days posttransplantation in untreated, control islet autograft recipients (Figure 1C). Figure 1Open in figure viewerPowerPoint Serial fasting blood glucose levels (FBS) and c-peptide levels in monkey islet autograft recipients and serial intravenous glucose tolerance tests (IVGTTs) in comparing a representative control and an alpha-1-antitrypsin (AAT)-treated autograft monkey recipient. (A) Blood glucose concentrations were measured daily, after overnight fasting in an untreated monkey (representative of four untreated monkeys); (B) twice a week, serum c-peptide levels are displayed in an untreated monkey (representative of four untreated monkeys); (C) IVGTTs were performed in non-AAT-treated autograft recipients at days 50, 90, 180 and 220 posttransplantation showing data representative of that obtained in four independent experiments. For comparison, IVGTTs were performed in a healthy control monkey (red triangle) and a fully diabetic monkey (green square). (D) Blood glucose concentrations were measured in an AAT-treated monkey (representative of five treated monkeys); compared with the other four control monkeys with islet autografts infused into the portal circulation, AAT treatment significantly impacts long-term islet autograft survival (p = 0.001). (E) Serum c-peptide levels were analyzed in an AAT-treated monkey (representative of five treated monkeys). (F) IVGTTs were performed in an AAT-treated recipient (representative of that obtained in five AAT-treated, autograft recipients). As controls, IVGTTs were performed in a healthy control monkey (green triangles) and a fully diabetic monkey (red squares). The effect of short-term AAT treatment (60 mg/kg on days −1, 3, 7 and 14) was studied in nine monkeys. In each of the nine monkeys, receiving islets in the portal vein or underneath the renal capsule, blood glucose levels sharply decreased. In four of the nine AAT-treated monkeys, the islets were placed under the kidney capsule in hosts rendered diabetic by partial pancreatectomy and STZ. Blood glucose levels sharply decreased to less than 150 mg/kg within 24 h posttransplantation in all four of these AAT-treated recipients as compared to 4 days in non-AAT-treated controls (p = 0.003). Indeed, fasting blood glucose levels in AAT-treated autograft recipients hosts were not different from normal nontransplanted monkeys. Long-term function transplant was also followed in five AAT-treated recipients receiving autologous islets infused into the portal circulation. One normoglycemic AAT-treated recipient was sacrificed at 468 days posttransplantation for histological studies. In the other four AAT-treated hosts, blood glucose levels normalized within 24 h posttransplantation (Figure 1D) and blood glucose levels have remained less than 100 mg/dL throughout long follow-up periods, that is 700–1200 days in the four remaining monkeys. Compared with the other four control monkeys with islet autografts infused into the portal circulation, AAT-treatment significantly impacts long-term islet autograft survival (p = 0.001). To date none of the AAT-treated recipients has developed recurrent diabetes. As previously noted in this autograft model 4, circulating c-peptide levels rise in the early posttransplant period (Figure 1E). In contrast to the pattern noted in untreated islet autograft recipients shown above (Figure 1B), circulating c-peptide levels do not deteriorate over a long follow-up time in the AAT-treated subjects (Figure 1E). Instead, normal circulating c-peptide levels are maintained in the treated recipients throughout lengthy periods of follow-up (Figure 1E). Blood samples were then collected at 0, 1, 3, 5, 10, 15, 20, 25, 30, 45 and 60 min postglucose challenge for assessment of blood glucose and c-peptide levels. IVGTTs were performed on an untreated diabetic and a normal, nontransplanted monkey. For comparison IVGTTs were repeatedly performed on AAT-treated autograft recipients for up to 4 years posttransplantation (Figure 1F). Throughout this period IVGTTs obtained in the AAT-treated recipients are indistinguishable from the normal monkey but differ markedly from the untreated diabetic monkeys. After three consecutive fasting blood glucose values of greater than 300 mg/dL in islet autograft recipients, a blood glucose level seen only in untreated control recipients, these recipients were sacrificed. Islets were not detected by histological analysis of the liver in any of these control, sacrificed diabetic autograft recipients (50 sections/levels cut per liver). Previous reports document total loss of islet autografts as detected by histology in monkeys and dogs that develop diabetes 14, 16, 17. In a histologic analysis of a euglycemic AAT-treated recipient receiving autologous islets in the portal bed at day 468 posttransplantation (Figure 2), 12 islets were identified in 20 sections/levels per liver with hematoxylin and eosin (H&E) and 23 small islets were identified by insulin staining in nine sections. For example, an islet is noted in a small portal vein with mild fibrin deposition (Figure 2A: H&E 200× and 2B 400×). Islets in portal venules are identified through their strong insulin immunostaining and counterstained with hematoxylin (Figure 2C: 220× and 2D: 400×). Figure 2Open in figure viewerPowerPoint Histological examinations of islets from an alpha-1-antitrypsin-treated autograft recipient 468 days posttransplantation. Serial liver samples were fixed prior to sectioning and staining. The following was observed: (A) Islets present in a small portal vein with mild fibrin deposition. Micro-steatosis is evident. Hematoxylin and eosin (H&E), 200×; (B) Islet in a small portal vein with minimal fibrin deposition and hepatic micro-steatosis. H&E, 400×; (C) 200× and (D) 400× islets in small portal venules with strong insulin staining detected by immunohistochemistry. The sections were counterstained with hematoxylin. Transcriptional and systems biology signatures in AAT-treated and untreated recipients For comparison, pairs of monkeys bearing islets transplanted beneath the renal capsule that were: (i) AAT-treated, euglycemic monkeys (n = 4) and (ii) untreated monkeys (n = 4) that recently became diabetic were sacrificed at approximately the same number of days posttransplantation. To identify the transcriptional changes induced by AAT treatment, we performed genome-wide transcriptional analysis on islets obtained from untreated and AAT-treated recipients selected as described above. After normalization and preprocessing of gene expression data, a total of 264 transcripts were significantly differentially expressed lower confidence bound (LCB 1.5) of which 93% of transcripts are down-regulated in islets from AAT-treated hosts. A heatmap of only the top differentially expressed genes is shown (Figure 3). The extended analysis revealed that AAT treatment down-regulated expression of multiple genes linked to the cell-mediated immune response, most prominently several chemokines and chemokine receptors genes such as TGFβ3, FOXP1, ANGPT2, THY1, CCL21 and CCL11, and cell cycle regulation gene such as SOCS3. While transcriptional proofing used alone is part is an underpowered approach for the small sample size interrogated, we have blended bioinformatics approaches designed to effectively analyze small sample size and capture differentially expressed genes as described in the Materials and methods section. Figure 3Open in figure viewerPowerPoint Transcriptional profiling of autologous, nonhuman primate transplanted islets at ca. 100–120 days posttransplantation. Top differentially expressed genes identified in a supervised analysis using lower confidence bound of fold change 1.5. Two alpha-1-antitrypsin (AAT)-treated and two untreated recipients are studied. The columns represent the samples, and the rows represent the genes. Gene expression is shown with pseudo color scale (−3 to 3) with red denoting high expression level and green denoting low expression level of gene. To understand the underlying biology of AAT treatment effects, we performed functional categories and canonical pathways analysis using the Ingenuity package. The functional analysis of differentially expressed genes identified significantly impacted categories (p 0.05) that include cellular movement, cell death and survival, cell cycle, connective tissue development, cell-mediated immune response and immune cell tracking. Most of the genes in these functional categories are significantly down-regulated by AAT and the results highlight the anti-inflammatory and anti-apoptotic properties of AAT (Figure 4). Furthermore, pathways analysis generated a list of differentially expressed genes identified significant pathways (p 0.05) that includes Tight Junction signaling, WNTβ catenin signaling, Integrin Linked Kinase (ILK) signaling, Glutathione redox reaction, Leukocyte extraversion signaling, Epithelial-Mesenchymal Transition (EMT) signaling and Paxillin signaling (Figure 4). To identify the biological networks perturbed in this complex, AAT treatment driven, islet signature, we performed system biology oriented analysis on 264 transcripts. The results show a clear effect of AAT treatment in which nine interactive networks of genes were identified that achieved a score ≥ 20. The network score is an indicator of significance; the higher the score, the more significantly the biological network is perturbed. A score of only 5 indicates that there is a 1/100 000 chance that the focus genes are in the network due to random chance. To understand the underlying biological mechanism, we merged significant networks with related functions and generated a comprehensive systems-level view of therapeutic changes induced by AAT (Figure S1). Merging the networks generated two super networks linked to the Immune Response and Connective Tissue (Figure S1A) and Cell Cycle and Proliferation related networks (Figure S1B). The interactive network-based analysis incorporated many genes in the network that are not significantly altered by AAT treatment at the transcriptional level (shown in white color), but the protein (i.e. gene product) might be altered at posttranscriptional or activity levels as deduced by the systems biology analysis. Many of these genes formed regulatory or highly connected nodes that serve as focus hubs in the networks. Merged network focus hubs identified are formed by inflammation-related molecules (e.g. NFκB), kinases (e.g. AKT, ERK), and cell proliferation-related genes (TGFβ). Similarly merging cell cycle, cellular proliferation and development networks generated a complex network with regulatory hubs formed by kinases (e.g. MAPK, JNK, p38 MAPK), inflammation-regulated genes (e.g. VEGF-C, pro-inflammatory cytokines complex) and cell cycle proliferation related genes (e.g. UBC, APP). The focus hubs forming genes are considered the most critical for overall function of the network. Interestingly, interactive network-based analysis identified the significant hubs formed by TGFβ, NFκB and AKT, supporting the hypothesis that AAT treatment affects the expression of genes whose critical focus hubs are formed by the NFκB pathway and AKT to produce a dramatic effect on islet survival as noted by histological examination in concert with the loss of inflammation. Figure 4Open in figure viewerPowerPoint Functional and pathways enrichment analysis of differentially expressed genes. (A) Significantly affected functions in posttransplant alpha-1-antitrypsin (AAT)-treated islets at ca. 100–120 days posttransplantation. About 8–100% of the genes in the functions are down-regulated by AAT treatment. (B) Significantly affected pathways in 3-day posttransplant AAT-treated islets. We have previously demonstrated that following subtotal pancreatectomy and destruction of remnant islets by STZ, transplantation of a marginal mass of autologous islets, islets harvested from 70% to 80% of a partial pancreatectomy specimen, leads to inexorable, insidious loss of graft function 4. Islet harvesting and transplant procedures are associated with an outpouring of inflammatory cytokines and one dose of AAT enables short-term graft function, albeit not permanent, of a very small syngeneic islet transplant mass in mice 12. Hence, we have now tested the ability of short-term peri-transplant AAT treatment, a potent inhibitor of pro-inflammatory cytokine expression 7, 9 to protect a marginal mass of monkey islet autografts from long-term, nonimmunological graft loss. While recurrence of diabetes and loss of the islets was observed in each untreated islet autograft recipient, independent of weight or age, by 180 days posttransplantation, none of the AAT-treated monkeys lost graft function throughout the long follow-up observation periods, that is, up to 4 years. We have previously demonstrated that 70–80% subtotal pancreatectomy also leads to slow beta cell failure 4. In this model, the islets are not manipulated and remain in situ within the native pancreas so that the fate of a marginal mass of islets can be more clearly studied apart from other variables. These experiments re-enforce the view that the presence of a very marginal islet transplant mass leads to nonimmunological islet loss (\"exhaustion”). A substantial portion of human islet autografts gradually lose function even after sustained periods of initial excellent function 18. The rate of autograft failure is particularly high when used as a means to treat patients with pancreatitis but transplantation of autologous islets in patients not afflicted with clinically evident pancreatitis is also subject to a substantial rate of failure 1. Moreover, an unexpectedly high rate of human islet allograft failure at 5 years posttransplantation is noted despite a high rate of successful engraftment at 1 year 1 in patients only given conventional immunosuppression. The mechanisms accounting for this high rate of autologous and allogeneic graft failure are poorly understood. Taken together islet transplants can and do fail as a result of nonimmunological mechanisms. Knowing that the loss of islets in the peri- transplant period from nonimmunologic means is massive, the full promise of islet cell transplantation in the clinic cannot be realized in the absence of engraftment of a large number of islets that survive the peri-transplant period. We have now tested the hypothesis that nonimmunologic loss of autologous islets can be drastically reduced by AAT. We hypothesized that administration of AAT, an acute phase reactant, that quenches adverse inflammation 19 would protect autologous islets from destruction and thereby help preserve euglycemia long-term. In our analysis of 17 autologous cynomolgus islet transplants, the dramatic effect of AAT on the long-term survival of islet autografts is self-evident. Islet graft failure was not evident in any recipients treated short term with AAT throughout a 4-year follow-up period (at which point the recipients were sacrificed). Indeed islet function increased over time in AAT-treated hosts. In contrast, all untreated control recipients became diabetic by 6 months posttransplantation. To gain insight as to the mechanism by which AAT promotes long-term survival, we placed autografts beneath the renal capsule in four control and four AAT-treated monkeys. Unlike islets scattered throughout the portal circulation, islet placed beneath the renal capsule are readily accessible for mechanistic studies. We acknowledge that islets placed in the portal bed and those under the renal capsule may subject to different insults, for example, instant blood-mediated inflammatory reaction. Hence, histologic and expression profile-based analysis of subcapsular islet transplants cannot be applied with absolute confidence to the unstudied intra-portal bed transplanted islets. When an autograft in a control monkey began to fail, genome-wide transcriptional profiles were obtained from grafts of a control and an AAT-treated host transplanted at approximately the same time point. Histopathology revealed viable islets in an AAT-treated but are not in a control recipient. In AAT-treated hosts, a series of immuno-inflammatory genes and pathways were muted. In this genome-wide expression profile and network-based analysis we now note for the first time that cell survival and death pathways were favorably impacted by AAT treatment. We acknowledge that conventional expression profile analysis applied to the small sample size used in the project would be underpowered, but our analysis was conducted with methods that include but not confined to network-based and PLIER algorithm analyzed bioinformatics. This approach, as described in more detail in the Materials and Methods section, was designed and proven to be informative when applied to small samples and accurately capture differentially expressed genes. We have described a similar transcriptional/bioinformatics profile in immune tissue and in insulin-sensitive tissues of AAT-treated nonobese diabetic (NOD) mice, mice that are afflicted with both T1D and T2D 19 as well as in a study analyzing the ability of AAT to protect very small syngeneic mouse islet transplants from immediate destruction posttransplantation 12. In consideration of the similarity our previous analysis of AAT effects upon inflammatory pathways in other models as well as bio-ethical and financial issues, we were dissuaded from pursuing a subsequent series of validation type bioinformatics experiments in the monkey model. Seventeen monkeys were studied to obtain the data in this report. In short, AAT dampens cytodestructive inflammation and facilitates healing and repair of islet transplants from the challenges that attend organ harvest, in vitro manipulation and in the period preceding neo-vascularization. The effects of AAT upon inflammation in the NOD model and, now seen in monkey islet transplant recipients, center on, but are not limited to, muting activation of the NFκB pathway as deduced by the notable impact upon its upstream as well as downstream pathway-related molecules and favorably alter expression of cell death and survival pathways as detected by a system biology approach and histology. These effects enabled functional expansion of the islet mass in transplanted hosts such that graft function improves rather than deteriorates over time. As AAT dramatically protects very small monkey islet autografts from immediate destruction and graft failure, short-term AAT treatment may prove useful in human transplantation. It is notable that the use of AAT as a replacement therapy in humans is safe 20. We thank Neal R. Smith, from the Massachusetts General Hospital, for his expert pathologic studies. This work was funded in part by NIH 5 RO1 AI50987, NIH 1-U19 DK080652, JDRF 7-2005-1329 (TBS and MK) and a JDRF postdoctoral fellowship grant (MB). The authors of this manuscript have no conflicts of interest to disclose as described by the American Journal of Transplantation. Additional Supporting Information may be found in the online version of this article. ajt12723-sm-0001-SuppFig-S1.pdf231.4 KB Figure S1: Network analysis of AAT regulated genes in islets. (A) Merged network of connective tissue and immune system related network. (B) Merged network of cell development, growth and movement-related networks. The Ingenuity Pathways Analysis (IPA) tool was used to generate the networks from the differentially expressed genes. The intensity of the node color indicates the degree of up-regulation (red) and down-regulation (green) in islets from AAT as compared to control recipient host. The core regulatory genes of the network are shown in bold line in the center of network. ajt12723-sm-0001-SuppTab-S1.pdf218.3 KB Table S1: Summary for the purity, viability, total islet numbers and IEQ of prepared islets for transplantation. The purity, viability and IEQ were analyzed on days 1 and 0 (day of the transplant). This table reflects the results from the day of islet transplantation. These methods have been our standard practice for the past 20 years in nonhuman primate islet transplant experiments 13. We found about 10% of our islets undergo apoptosis and die in the overnight culture period. Please note: The publisher is not responsible for the content or functionality of any supporting information supplied by the authors. Any queries (other than missing content) should be directed to the corresponding author for the article.Korsgren O. Isolated human islets trigger an instant blood mediated inflammatory reaction: implications for intraportal islet transplantation as a treatment for patients with type 1 diabetes. Ups J Med Sci 2000; 105: 125– 133.Smith RN. The effect of low versus high dose of streptozotocin in cynomolgus monkeys (Macaca fascicularis). Am J Transplant 2003; 3: 267– 272.Strom TB. Prolonged survival of allogeneic islets in cynomolgus monkeys after short-term anti-CD154-based therapy: Nonimmunologic graft failure? Am J Transplant 2006; 6: 687– 696.Dammin GJ. Immunologic enhancement of rat renal allografts. III. Immunopathologic lesions and rejection in long-surviving passively enhanced grafts. Am J Pathol 1975; 79: 255– 270.Zay K, et al. Alpha-1-antitrypsin and a broad spectrum metalloprotease inhibitor, RS113456, have similar acute anti-inflammatory effects. Lab Invest 2001; 81: 1119– 1131.Zhu C. Protective effects of alpha 1-antitrypsin on acute lung injury in rabbits induced by endotoxin. Chinese Med J 2003; 116: 1678– 1682.Dinarello CA. Alpha1-antitrypsin monotherapy prolongs islet allograft survival in mice. Proc Natl Acad Sci U S A 2005; 102: 12153– 12158.Zhen L, et al. A novel antiapoptotic role for alpha1-antitrypsin in the prevention of pulmonary emphysema. Am J Respir Crit Care Med 2006; 173: 1222– 1228. Brantly M. Alpha1-antitrypsin: Not just an antiprotease: Extending the half-life of a natural anti-inflammatory molecule by conjugation with polyethylene glycol. Am J Respir Cell Mol Biol 2002; 27: 652– 654.Fan Z, et al. Alpha 1-antitrypsin reduces inflammation and enhances mouse pancreatic islet transplant survival. Proc Natl Acad Sci U S A 2012; 109: 15443– 15448.Parent RJ, et al. A simple and cost-effective method for the isolation of islets from nonhuman primates. Cell Transplant 2003; 12: 883– 890.Huber W. arrayQualityMetrics—A bioconductor package for quality assessment of microarray data. Bioinformatics 2009; 25: 415– 416.Hoffman L, et al. Curative and beta cell regenerative effects of alpha1-antitrypsin treatment in autoimmune diabetic NOD mice. Proc Natl Acad Sci U S A 2008; 105: 16242– 16247.Campos M. Safety and efficacy of alpha-1-antitrypsin augmentation therapy in the treatment of patients with alpha-1-antitrypsin deficiency. Biolog: Targets Ther 2009; 3: 193– 204. The full text of this article hosted at iucr.org is unavailable due to technical difficulties. Please check your email for instructions on resetting your password. If you do not receive an email within 10 minutes, your email address may not be registered, and you may need to create a new Wiley Online Library account. Can\'t sign in? Forgot your username? Enter your email address below and we will send you your username If the address matches an existing account you will receive an email with instructions to retrieve your username
本文链接: https://www.ebiomall.cn/b328-spl/info-37860.html
免责声明 本文仅代表作者个人观点,与本网无关。其创作性以及文中陈述文字和内容未经本站证实,对本文以及其中全部或者部分内容、文字的真实性、完整性、及时性本站不做任何保证或承诺,请读者仅作参考,并请自行核实相关内容。
版权声明 未经蚂蚁淘授权不得转载、摘编或利用其他方式使用上述作品。已经经本网授权使用作品的,应该授权范围内使用,并注明“来源:蚂蚁淘”。违反上述声明者,本网将追究其相关法律责任。
相关文章
2016-05-25
2016-10-19
2017-10-27
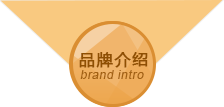

▍
品牌分类
暂无品牌分类
▍
官网分类
▍
品牌问答