新闻动态
The absence of HLA class I expression in non‐small cell lung...
2025-06-15
The absence of HLA class I expression in non‐small cell lung cancer correlates with the tumor tissue structure and the pattern of T cell infiltration - Perea - 2017 - International Journal of Cancer - Wiley Online LibraryInternational Journal of CancerVolume 140, Issue 4 p. 888-899 Tumor Immunology and Microenvironment Free Access The absence of HLA class I expression in non-small cell lung cancer correlates with the tumor tissue structure and the pattern of T cell infiltration Francisco Perea, Servicio de Análisis Clínicos e Inmunología, UGC Laboratorio Clínico; Hospital Universitario Virgen de las Nieves, Granada, SpainSearch for more papers by this authorMónica Bernal, Servicio de Análisis Clínicos e Inmunología, UGC Laboratorio Clínico; Hospital Universitario Virgen de las Nieves, Granada, SpainSearch for more papers by this authorAbel Sánchez-Palencia, Servicio de Cirugía Torácica, Hospital Universitario Virgen de las Nieves, Granada, SpainSearch for more papers by this authorJavier Carretero, Servicio de Análisis Clínicos e Inmunología, UGC Laboratorio Clínico; Hospital Universitario Virgen de las Nieves, Granada, SpainSearch for more papers by this authorCristina Torres, Servicio de Análisis Clínicos e Inmunología, UGC Laboratorio Clínico; Hospital Universitario Virgen de las Nieves, Granada, SpainSearch for more papers by this authorClara Bayarri, Servicio de Cirugía Torácica, Hospital Universitario Virgen de las Nieves, Granada, SpainSearch for more papers by this authorMercedes Gómez-Morales, Departamento de Anatomía Patológica, Universidad de Granada, Granada, SpainSearch for more papers by this authorFederico Garrido, Servicio de Análisis Clínicos e Inmunología, UGC Laboratorio Clínico; Hospital Universitario Virgen de las Nieves, Granada, Spain Instituto de Investigación Biosanitaria ibs.Granada, Granada, Spain Departamento de Bioquímica, Biología Molecular e Inmunología III, Universidad de Granada, Granada, SpainSearch for more papers by this authorFrancisco Ruiz-Cabello, Corresponding Author fruizc@ugr.es Servicio de Análisis Clínicos e Inmunología, UGC Laboratorio Clínico; Hospital Universitario Virgen de las Nieves, Granada, Spain Instituto de Investigación Biosanitaria ibs.Granada, Granada, Spain Departamento de Bioquímica, Biología Molecular e Inmunología III, Universidad de Granada, Granada, SpainCorrespondence to: Francisco Ruiz-Cabello, Servicio de Análisis Clínicos e Inmunología, Hospital Virgen de las Nieves, Av. Fuerzas Armadas s/n, Granada, Spain, Tel.: +34-958020320, Fax: +34-958020120, E-mail: fruizc@ugr.esSearch for more papers by this author Francisco Perea, Servicio de Análisis Clínicos e Inmunología, UGC Laboratorio Clínico; Hospital Universitario Virgen de las Nieves, Granada, SpainSearch for more papers by this authorMónica Bernal, Servicio de Análisis Clínicos e Inmunología, UGC Laboratorio Clínico; Hospital Universitario Virgen de las Nieves, Granada, SpainSearch for more papers by this authorAbel Sánchez-Palencia, Servicio de Cirugía Torácica, Hospital Universitario Virgen de las Nieves, Granada, SpainSearch for more papers by this authorJavier Carretero, Servicio de Análisis Clínicos e Inmunología, UGC Laboratorio Clínico; Hospital Universitario Virgen de las Nieves, Granada, SpainSearch for more papers by this authorCristina Torres, Servicio de Análisis Clínicos e Inmunología, UGC Laboratorio Clínico; Hospital Universitario Virgen de las Nieves, Granada, SpainSearch for more papers by this authorClara Bayarri, Servicio de Cirugía Torácica, Hospital Universitario Virgen de las Nieves, Granada, SpainSearch for more papers by this authorMercedes Gómez-Morales, Departamento de Anatomía Patológica, Universidad de Granada, Granada, SpainSearch for more papers by this authorFederico Garrido, Servicio de Análisis Clínicos e Inmunología, UGC Laboratorio Clínico; Hospital Universitario Virgen de las Nieves, Granada, Spain Instituto de Investigación Biosanitaria ibs.Granada, Granada, Spain Departamento de Bioquímica, Biología Molecular e Inmunología III, Universidad de Granada, Granada, SpainSearch for more papers by this authorFrancisco Ruiz-Cabello, Corresponding Author fruizc@ugr.es Servicio de Análisis Clínicos e Inmunología, UGC Laboratorio Clínico; Hospital Universitario Virgen de las Nieves, Granada, Spain Instituto de Investigación Biosanitaria ibs.Granada, Granada, Spain Departamento de Bioquímica, Biología Molecular e Inmunología III, Universidad de Granada, Granada, SpainCorrespondence to: Francisco Ruiz-Cabello, Servicio de Análisis Clínicos e Inmunología, Hospital Virgen de las Nieves, Av. Fuerzas Armadas s/n, Granada, Spain, Tel.: +34-958020320, Fax: +34-958020120, E-mail: fruizc@ugr.esSearch for more papers by this author Give accessShare full text accessShare full-text accessPlease review our Terms and Conditions of Use and check box below to share full-text version of article.I have read and accept the Wiley Online Library Terms and Conditions of UseShareable LinkUse the link below to share a full-text version of this article with your friends and colleagues. Learn more.Copy URLShare a linkShare onEmailFacebookTwitterLinked InRedditWechat Abstract We wanted to analyze whether tumor HLA class I (HLA-I) expression influences the pattern of the immune cell infiltration and stromal cell reaction in the tumor microenvironment. Tumor tissues obtained from 57 patients diagnosed with lung carcinomas were analyzed for HLA expression and leukocyte infiltration. 28 patients out of the 57 were completely negative for HLA-I expression (49.1%) or showed a selective HLA-A locus downregulation (three patients, 5.2%). In 26 out of 57 tumors (47.8%) we detected a positive HLA-I expression but with a percentage of HLA-I negative cells between 10 and 25%. The HLA-I negative phenotype was produced by a combination of HLA haplotype loss and a transcriptional downregulation of β2-microglobulin (β2-m) and LMP2 and LMP7 antigen presentation machinery genes. The analysis and localization of different immune cell populations revealed the presence of two major and reproducible patterns. One pattern, which we designated \"immune-permissive tumor microenvironment (TME),” was characterized by positive tumor HLA-I expression, intratumoral infiltration with cytotoxic T-CD8+ cells, M1-inflammatory type macrophages, and a diffuse pattern of FAP+ cancer-associated fibroblasts. In contrast, another pattern defined as \"non-immune-permissive TME” was found in HLA-I negative tumors with strong stromal-matrix interaction, T-CD8+ cells surrounding tumor nests, a dense layer of FAP+ fibroblasts and M2/repair-type macrophages. In conclusion, this study revealed marked differences between HLA class I-positive and negative tumors related to tissue structure, the composition of leukocyte infiltration and stromal response in the tumor microenvironment. Abbreviations APM antigen processing machinery CTL cytotoxic T lymphocyte FAP fibroblast activation protein HC heavy chain HLA human leukocyte antigen LOH loss of heterozygosity LOT lymphocyte outside the tumor mAbs monoclonal antibodies MHC major histocompatibility complex NSCLC non-small-cell lung carcinoma PBL peripheral blood lymphocyte PD-1 Programmed death-1 PDL-1 Programmed death-ligand-1 SCC squamous cell carcinomas SPSS Statistical Package for the Social Sciences TIL tumor infiltrating lymphocyte TME tumor microenvironment TNM tumor-node-metastasis β2-m beta-2-microglobulin Leukocytes and especially T lymphocytes can infiltrate tumor tissue independently of the histological type. This infiltration has been described in melanoma,1 esophageal,2 colorectal,3-5 pancreatic,6 ovarian,7 gallbladder,8 breast,9 prostate,10 and lung carcinomas.11 Recently, attention focused on the type, density and location of leukocyte infiltration in human tumors and its impact on prognosis. The clinical significance of a detailed analysis of the cellular infiltration (called \"immunoscore”) appeared to be greater than that of TNM tumor classification.12 However, the factors that pre-determine the different patterns of tumor infiltration are not known. Some tumor tissues have different types of leukocytes, including CD8+ T cells, which surround tumor cells and are restricted exclusively to the peritumoral-stroma and the invasive margin. In contrast, in other tumors, the presence of CD8+ tumor-infiltrating T lymphocytes is observed within tumor islets. Recently, it has been proposed that the matrix architecture contributes to these distinct patterns of infiltration and migration of T cells into the stroma, preventing direct contact with tumor cells and contributing to immune evasion.13, 14 The reason why the tumor tissue architecture changes so dramatically from the infiltration to the non-infiltration stage is not known. A high proportion of human tumors can evade T lymphocyte responses by losing or downregulating the expression of HLA-I molecules.15-17 This immune escape mechanism is well documented and has been reported and confirmed by different groups.18-20 Total or selective HLA-A,B,C losses have been reported in a large variety of human tumors derived from HLA-I positive epithelia.21-23 The molecular mechanism responsible for HLA-I losses is crucial for the possible restoration of the expression of these molecules. If the molecular mechanism is reversible (soft), HLA-I molecules will be recovered after the release of TH1 type cytokines in the TME and the tumor will be rejected by CTLs.24 In contrast, if the mechanism is irreversible due to structural alterations in HLA-I or β2-m genes (hard lesions), the HLA-I expression will not be recovered and the tumor will continue growing and will eventually kill the host.24 In this paper we present evidence of a direct correlation among patterns of tumor HLA-I expression, patterns of tumor infiltrating lymphocytes/macrophages, and the tissue stroma/matrix architecture in lung cancer. When the tumor is HLA-I positive CD45RO, CD3, and CD8-positive T lymphocytes, as well as CD64 and CD206-positive macrophages are in close contact with tumor cells inside the tumor nests. In contrast, these cells are in the stroma in HLA-I negative tumors and outside the tumor tissue. Interestingly, we found that the presence of these two patterns of stromal reaction correlates with the ratio of macrophage M1/M2 polarization. 57 NSCLC (Non-small cell lung cancer) patients were included in this study. Patient samples were obtained from Virgen de las Nieves University Hospital (Granada, Spain). Demographic, clinical and histological characteristics of studied subjects are summarized in Table 1. Before the study, all medical records and tumor sections were reviewed by an oncologist and a surgical pathologist. Informed consent was obtained from all the patients, which was approved by the Ethics Committee of our institution. The specimens included 30 squamous cell carcinomas (SCC), 25 adenocarcinomas and two large cell carcinomas based on WHO criteria of histopathological classification.25 The tumors were classified as stage I (n = 30), and stage II and III (n = 24) based on the American Joint Committee on Cancer guidelines for postsurgical, tumor-node-metastasis (TNM).26 Five cases received radiation or chemotherapy before surgery. 57 NSCLC tumors were analyzed in the present study, including 40 cryopreserved and 17 paraffin embedded tissues. Tumor samples were taken from primary malignant lung tumors by excision of a fragment of tumor mass during the initial surgery for the disease. After thoracotomy and lung resection, half of the piece was immediately stored at −80°C. Frozen tumor tissue sections of 4–8-μm-thick tissues were cut on a microtome-cryostat (Bright), allowed to dry at room temperature for 4–18 hr, fixed in acetone at 4°C for 10 min, and stored at −40°C until staining. The other half of the piece was fixed in 10% neutral formalin and embedded in paraffin. 4 μm-thick sections were mounted on pretreated slices. Immunohistological techniques were performed with the Biotin-Streptavidin System (supersensitive Multilink HRP/DAB kit, BioGenex, The Hague, The Netherlands). The following mouse monoclonal antibodies (mAbs) were used in cryopreserved tumor tissues to analyze HLA-I expression: W6/32 against HLA-A, B, and C heavy chain/β2-m complex (a gift from Dr Bodmer, Imperial Cancer Research Fund Laboratories, London, UK); GRH-1, which recognizes free and HLA class I heavy chain-associated β2-m chain, produced and characterized in our laboratory; HC-10 against free heavy chain of HLA-B and C molecules, anti-HLA-A which recognizes a subset of HLA-A locus-encoded gene products and 42IB5 against HLA-B locus-encoded gene products. We also studied HLA class II expression by using GRB1 mAb against HLA-DR molecules, produced and characterized in our laboratory. These mAbs were previously reported.5, 10 Total loss of HLA-I molecules was defined by negative staining withW6/32 and GRH-1 mAbs according to the criteria established by the HLA and Cancer component of the 1996 International Histocompatibility Workshop.27 Paraffin embedded tissues were stained for EMR8-5 against HLA-ABC heavy chain (Clone ab70328, abcam), L-368 which recognizes β2-m chain (a gift from Dr. Ferrone, Harvard University) and HC-10, specific for HLA-B and C free heavy chain.5 Before immunolabeling paraffin sections were deparaffinized and endogenous peroxidase activity blocked using a solution of hydrogen peroxide for 10 minutes. For antigen retrieval, sections were treated with sodium citrate buffer (pH 6.0). Mouse mAbs used to stain tumor infiltrates in cryostatic sections were: GRT2 (anti-CD45), produced in our laboratory,5 OKT3 (anti-CD3, ATCC, Teddington, UK), OKT8 (anti-CD8, ATCC), anti-CD45RO (Clone UCHL1, Thermo Fisher), anti-CD64 (Clone 10.1, BD Biosciences), anti-CD206 (Macrophage mannose receptor; Clone 19.2, BD Biosciences), anti-CD56 (Clone 123C3, Dako) and an anti-FAP (Fibroblast Activation Protein), (Clone F11-24, Merck Millipore). Paraffin-embedded tissue sections were also stained for CD45, CD4, CD8, using prediluted antibodies supplied by Master Diagnóstic (CD45: Ref MAD-002066QD, batch:0108–01; CD4: Ref: MAD-00600QD, batch:0107–02-10, clone EP 204; CD8: Ref MAD-000618QD, batch 0183–11, clone SP16. Immunohistochemical staining was carried out using the of UltraVision Quanto (peroxidase) immunohistochemistry detection system (MAD-021881QK/MAD-001881QK Master Diagnóstica) following manufacturer\'s instructions. The primary antibody was replaced with PBS for negative controls; no immunohistochemical staining was detected in control preparations. Three observers analyzed tissue tumor infiltrates, using 10x and 40x objectives and defining the tumor infiltration using anti-CD45, anti-CD8 and anti-CD64 mAbs as follows: (1) tumor infiltration with lymphocytes (TILs) and macrophages along with stromal infiltration. TILs were observed in close contact with neoplastic epithelial cells (20–70 TILs per field at 40× magnification) and (2) stromal infiltration (inflammatory infiltrate only encapsulating tumor mass). Global infiltration by CD45, CD3, CD8, CD45RO and CD64 positive cells was evaluated in 5 different fields under 40× objective and scored as: + (≤ 30 cells/field), ++ (30–70 cells/field), +++ (70–120 cells/field) and ++++ (≥120 cells/field). For each cryopreserved tumor sample we calculated the infiltration score (1–4) for each marker (CD45, CD3, CD8, CD45RO and CD64) by adding one point for each assigned cross (+) for subsequent statistical analysis. Macrophage subpopulations were studied using mAbs against CD64 (M1 or inflammatory macrophage marker) and CD206 (M2 or immunosuppressive macrophage marker). Tissue sections for staining with both mAbs were scanned using Pannoramic MIDI Scanner (3DHISTECH). For each tumor sample, M1 and M2 subpopulations were quantified in five different fields by two independent observers using Viewer MIDI software and 40× objective, and subsequently the M1/M2 ratio was calculated in each case. All statistical analyses were performed using the Statistical Package for the Social Sciences (SPSS), version 20.0. Variables with normal distribution are expressed as means with standard deviation. Variables with non-normal distribution are expressed as medians and interquartile range. In the case of quantitative variables, differences among all study groups were evaluated by using a t test in case of normality or a non- parametric test (Wilcoxon or Mann Whitney Test) for non-normal variables, when two groups were compared. To check for normality we used Shapiro Wilk and Kolmogorov-Smirnov tests. Categorical variables, such as sex, smoking history, tumor stage, HLA-I expression were coded in two groups and analyzed using the χ2 (X2) or Fisher\'s exact test. Differences were considered statistically significant at p 0.05. Cryopreserved tissue sections 4–8-μm-thick, fixed in 70% ethanol and stained with a 0.05% w/v solution of toluidine blue, were microdissected using a laser micromanipulator (PALM MicroLaser Systems, ZEISS). Microdissected fragments were collected in PALM Adhesive Caps. Tumor DNA was isolated from microdissected tumor fragments using QIAamp Tissue Kit (QIAGEN, the Netherlands). In all cases, normal autologous DNA was obtained from peripheral blood lymphocytes (PBLs), also using the QIAGEN DNA isolation kit following the manufacturer\'s protocol. DNA and RNA obtained from HLA-I negative tumors and controls (PBLs) were evaluated for B2M gene sequencing, HLA-I genomic typing, loss of heterozygosity (LOH) analysis and gene expression study by RT-PCR (see Supporting Information Material and Methods) The expression of HLA-I antigens was evaluated in 57 primary lung cancer tissue samples using immunohistochemical staining with monomorphic determinants of HLA-ABC and locus-specific and anti-β2-m antibodies. Leukocytes and other interstitial cells revealed a strong cell surface HLA-I expression. However, we detected various HLA-I alterations in 32 out of 57 samples (56.1%) (Table 2 and Supporting Information Table S1). Table 2. Immunohistochemical analysis of HLA expression and leukocyte infiltration in frozen tissues of lung cancer NOTE 1 ‘‘–’’ 75–100% negative tumor cells, ‘‘+’’ 75% positive tumor cells, \"H” Heterogeneous, 25–75% positive tumor cells, \"N” No results. 2We show the global tumor infiltrate by leukocytes (CD45), including T lymphocytes (CD3), cytotoxic T cells (CD8), activated memory lymphocytes (CD45RO) and macrophages (CD64). The tumor infiltration is classified as follows: + (≤ 30 cells/field), ++ (30–70 cells/field), +++ (70–120 cells/field) and ++++ ( 120 cells/field). We also classify tumor infiltration by lymphocytes (TILs) (CD3 + CD8+) as follows: \"+” (20–70 TILs/field); \"+/–” (≤ 20 TILs/field); \"–” (absence of TILs). Absence or few isolated CD56+ NK cells was observed in tumors. The most frequent alteration detected in cryopreserved sections was total loss or downregulation of HLA-ABC molecules, which was observed in 22 out of 40 cases (55%) (Table 2). These alterations affected both HLA heavy and light chains, since no immunolabeling was detected when either anti-HLA-ABC-β2-m complex (W6/32) or anti-β2-m (GRH-1) antibodies were used. Interestingly, in 9 cases out of 22 (40.9%), we observed positive intracellular labeling of free heavy chains (HC-10 mAb positivity). In 3 tumor samples (7.5%) we detected a selective loss of HLA-A locus expression. In 15 out of 40 tumors (37.5%) the HLA-I expression was positive, but with the presence of 10–25% of HLA-I negative cells. Only 17 paraffin embedded samples were available (Supporting Information Table S1); out of which 6 tumors (35.3%) showed a complete loss of HLA-I expression. For a more detailed HLA analysis, we followed the protocol suggested by the HLA and Cancer component of the XII International Histocompatibility Workshop.27 We also studied the HLA class II expression using the monoclonal antibody against HLA-DR (GRB-1); 32 out of 40 cryopreserved samples (80%) were negative and six were positive for HLA-DR. These results are presented in Table 2. Table 1 summarizes different clinico-pathological parameters (histology, pathological stage, nodal status). No significant association was found between HLA-I expression and any of the parameters analyzed. In 40 frozen tumor samples, we analyzed the composition and localization of the tumor leukocyte infiltration, especially of cytotoxic T cells. For this purpose, we used mAbs against molecules expressed by leukocytes (CD45), cytotoxic T cells (CD3 and CD8), activated memory lymphocytes (CD45RO), M1 (CD64) and M2 (CD206) macrophages, and NK cells (CD56). Lymphocyte/macrophage infiltration was classified into two patterns based on their localization: (a) pattern with immune cells mainly present in stromal tissue and low or moderate intratumoral infiltration by lymphocytes (TILs) and macrophages; (b) stromal pattern when lymphocytes/macrophages reside only outside tumor nests, encapsulating them. We observed that all of the tumors with HLA-I total loss showed a stromal pattern with absence or very low numbers of TILs. In 16 out 22 tumors (72.7%) with HLA-I total loss, we detected a stromal pattern with absence of HLA-I positive cells within the tumor. In these cases, all positive cells for leukocyte/lymphocytes/macrophage markers were outside the tumor islet, in the stromal tissue. In 5 HLA-I negative tumors (22.7%), we observed a very low presence of CD8+ T cells inside tumor nests (≤20 TILs per field at 40× magnification). Only one HLA-I negative tumor (P11) showed a moderate presence of TILs (20–70 TILs per field at 40× magnification). Interestingly, this tumor was also positive for DR expression (Table 2). In contrast, in tumors with HLA-I positive or heterogeneous immunolabeling, cellular infiltration was clearly detectable close to tumor cells and the tissue structure was diffuse, with a mixture of both tumor cells and lymphocytes/macrophages. Only one HLA-I positive tumor (P21) presented the stromal pattern observed in the HLA-I negative tumor group, suggesting the existence of a yet unknown HLA-associated tumor escape mechanism. Similarly, this absence of leukocytes (lymphocytes/macrophages) was also detected in 2 out of 3 tumors with selective loss of HLA-A locus expression (P20, P31) (Table 2), whereas the remaining case (P42) showed the presence of TILs. Once again, this case showed positive tumor HLA-DR expression (Table 2). The statistical analysis revealed an association between tumor HLA-I expression and tumor infiltration pattern, i.e., HLA-I total loss was associated with stromal pattern and HLA-I positive expression with the presence of TILs (p = 0.009) [Supporting Information Fig. S1(a)]. We named these two different situations as permissive and non-permissive TME (see Figs. 1 and 2 and Table 2). Positive HLA class I expression and CD8+ infiltration patterns in NSCLC tumors. Tumor tissue was stained with anti-HLA-class I and anti CD8 monoclonal antibodies. Most of the cancer cells were HLA-class I positive in both tumor samples, P4 (large cell carcinoma in stage I) (Figs. 1a and 1b) and P24 (squamous cell carcinoma in stage II) (Figs. 1c and 1c). HLA-I positive tumor cells and HLA-I positive immune cells are not clearly distinguishable from each other (Figs. 1a−1d). A significant CD8+ infiltration was observed both within tumor nests and in the tumor-surrounding stroma (Figs. 1b−1d). The tissue structure is \"permissive,” allowing TILs to enter the tumor mass and achieve direct contact with cancer cells. This pattern in HLA-I positive tumors differs from that observed in HLA-I negative tissues and described in Figure 2 (see below). Original magnification: Figure 1a ×40 (scale bar = 50 µm) and Figures 1b−1d ×20 (scale bar = 100 µm). Images in Figures 1b and 1d are obtained from paraffin-embedded tissues, and those in. Figures 1a and 1c from cryopreserved tissue samples. Negative HLA class I expression and CD8+ infiltration patterns in NSCLC tumors. In contrast to Figure 1, tumor tissues of patient P16 (squamous cell carcinoma in stage II) (Figs. 2a and 2b) and P60 (squamous cell carcinoma in stage I) (Figs. 2c and 2d) are HLA class I negative (Figs. 2a and 2c). The CD8+ cells are restricted almost exclusively to the peritumoral stroma that surrounds the tumor nest in a \"non-permissive” tissue zone. Original magnification: Figures 2a−2d ×20 magnification (scale bar = 100 µm). Figure 2a comes from cryopreserved and Figures 2b−2d from paraffin-embedded tissue samples. Furthermore, based on a previous study (10), we quantified the infiltration by leukocytes (CD45), including T lymphocytes (CD3), cytotoxic T cells (CD8), activated memory lymphocytes (CD45RO) and macrophages (CD64) in all cryopreserved tumor samples (Table 2). We found a higher infiltration by CD45RO positive cells in the HLA-I positive tumor group than in HLA-I negative samples (p = 0.007). CD56 mAb was used to study the presence of NK cells in cryopreserved tumor tissues. Complete absence or the presence of a few isolated NK cells was observed in both HLA-I positive and negative tumors. We also investigated the presence and distribution of FAP+ fibroblasts in the tumor environment in association with tumor HLA-I expression and immune cell infiltration. We observed that in the majority of HLA-I positive tumors with presence of TILs [8 out of 14 (57.1%)], FAP+ fibroblasts were mixed with tumor cells and lymphocytes/macrophages, forming a loose network without clearly defined tumor nests (Figs. 3a and 3b). In contrast, HLA-I negative samples showed a dense network of FAP+ fibroblasts isolating tumor nests with a stromal/encapsulated structure [14 out of 17 (82.3%)] (Figs. 4a and 4b). In this context, we found no statistically significant association between tumor tissue structure (HLA-negative/stromal/encapsulated tumors or HLA-positive/TILs tumors) and type of FAP+ fibroblast localization (p = 0.128). Tumor tissue architecture of HLA class I positive samples is correlated with greater infiltration by M1 macrophages. (a) P4 and (b) P37 are HLA-I positive tumors with presence of TILs. In these HLA-I positive samples, FAP+ fibroblasts are in close contact with neoplastic cells and leukocytes (lymphocytes/macrophages), forming a mixed tissue structure. HLA-I positive tumors with TIL/mixed structure show a predominant infiltration by M1 (CD64+) rather than M2 (CD206+) macrophages (mean ratio M1/M2 = 1.3). These results contrast with the data obtained from HLA-I negative tumors described in Figure 4 (see below) Original magnification: Figure 3a and 3b ×10 (scale bar = 200 µm). Tumor tissue architecture of HLA class I negative samples is correlated with a greater infiltration by M2 macrophages. (a) P16 and (b) P34 are HLA-I negative tumors with stromal infiltration. In contrast to HLA-I positive tumors (Fig. 3), FAP+ fibroblasts in these HLA-I negative tumors shape a dense network that encapsulates tumor nests. These HLA-I negative tumors with stromal/encapsulated structure show a higher presence of M2 (CD206+) than M1 (CD64+) cells (mean ratio M1/M2 = 0.72) (p = 0.005). The dense layer of FAP+ fibroblasts isolating tumor nests in HLA-I negative tumors is correlated with a prevalence of M2/type repair-immunosupressor macrophages. Original magnification: Figure 4a and 4b FAP staining ×5 (scale bar= 500 µm) and Figures 4a and 4b M1 and M2 staining ×10 (scale bar = 200 µm). We observed differences in the infiltration by macrophage subpopulations according to the localization of FAP+ fibroblasts in the tumor environment. All tumors with a mixed network of fibroblasts/tumor cells/leukocytes (predominantly HLA-I positive tumors with presence of TILs) showed a balanced presence of M1 and M2 macrophages or a higher infiltration by M1 cells (mean M1/M2 ratio= 1.3). In contrast, tumors with a dense network of fibroblasts around tumor nests (fundamentally HLA-I negative tumors with stromal/encapsulated structure) showed a predominance of M2 macrophages over M1 cells (mean M1/M2 ratio = 0.72) [Figs. 3 and 4 and Supporting Information Fig. S2(b)]. Significant differences in M1/M2 ratio were found between tumors with a dense network of fibroblasts and samples with a mixed network (p = 0.005). Thus, the pattern of FAP+ fibroblasts described in most HLA-I negative tumors with stromal/encapsulated structure is associated with a marked M2 macrophage polarization, whereas the infiltration by M1 cells is related to HLA-I positive tumors with a mixed network of fibroblasts/tumor cells/leukocytes. One of the objectives of our work was to investigate the molecular mechanisms involved in total loss of HLA-ABC in HLA-I negative tumors identified by the immunohistochemical study (Supporting Information Material and Methods). We performed a study of gene expression using tumor mRNA from five HLA-I-negative tumors using laser capture microdisection. mRNA from six PBL samples was used as Controls. We analyzed the expression of Heavy-Chain HLA-I (HC) genes, B2M genes, antigen processing machinery (APM) genes (TAP1, TAP2, Tapasin, LMP2, LMP7, Calnexin and Calreticulin) and NLRC5 genes involved in regulation of HLA-I expression. We found a strong downregulation of B2M gene in tumor tissues compared to PBL group (controls) (p 0.006) (Supporting Information Table S2). LMP2 and LMP7 gene mRNA levels were also reduced in lung tumors as compared to Controls (LMP2, p 0.008; LMP7, p 0.041). We also detected a lower expression of HC and TAP1 genes in tumor samples, but the difference with the control did not reach statistical significance. Similarly, the observed upregulation of TAP2, Tapasin, Calreticulin and Calnexin and NLRC5 in lung tumor samples was not statistically significant (Supporting Information Table S2). Furthermore, we performed HLA-I genomic typing using tumor and normal autologous DNA from 10 HLA-I negative lung tumors to evaluate the presence of HLA-I gene losses. We observed that 6 out of 10 tumors showed a hemizygosity pattern compatible with a HLA-I haplotype loss. Only one tumor retained six HLA-I alleles typed using normal autologous DNA, whereas in three other cases the results were not conclusive (Supporting Information Table S3). We confirmed these typing results with a LOH study using six STRs (Short Tandem Repeats) associated with HLA region (6p21). Tumors with HLA haplotype loss showed LOH in 1–4 microsatellite markers of chromosome 6. However, no LOH in chromosome 6 was detected in a tumor without haplotype loss (P25) (Supporting Information Table S3). We also included in this study two microsatellite markers flanking the B2M gene. We detected LOH in at least one marker flanking B2M gene in 5 out of 10 HLA-I negative tumors. Interestingly, we did not detect mutations affecting the B2M gene after sequencing exon 1 and 2 in 10 HLA-ABC-negative tumors (Supporting Information Table S3). Lung cancer (NSCLC) is one of the most immunogenic tumors, and multiple peptides derived from non-synonymous mutations can potentially be recognized by cytotoxic T-CD8 lymphocytes.28 However, malignant lesions attempting to escape immunosurveillance establish a complex immunosuppressive network that evolves towards the selection of low immunogenic HLA-I negative or deficient clones and culminates in complete resistance to T-cell attack.16, 19, 29, 30 No published data are available on the possible relationship between the immune contexture (density and localization of leukocyte infiltration) and tumor HLA-I expression. The present findings indicate that tumor HLA-I expression is strongly correlated with the lymphocyte/macrophage infiltration pattern and tumor tissue architecture and reveal how an immune-privileged compartment with ineffective anti-tumor immunity is generated in the development of lung cancer. We observed a significant percentage of lung tumors with total loss or down-regulation of HLA-I molecules. Moreover, HLA-I negative tumors could be correlated with a reproducible and particular distribution of different leukocytes and macrophages in stromal tissue. We reported in 1991 that lung cancer cells downregulated HLA-I antigens in 25% of the studied patients.31, 32 In the present series, the percentage of HLA-I deficient tumor samples rose to 56.1%, consistent with previous publications.33, 34 The reasons for the discrepancy in the percentage of alterations (i.e., tumors with HLA loss) between our previous study and the present investigation are unknown, although it may be attributable to the utilization of more sensitive immunohistochemistry methods or to changes in the epidermoid/adenocarcinoma ratio in the patient population. We observed that LOH at 6p21.3 is an important cause of HLA haplotype loss in a significant number of cases analyzed. Haplotype loss of HLA-I antigens was found in 40% of lung cancer cell lines,35, 36 and is one of the most common causes of abnormal HLA-I expression in various tumor types.18, 36 In this context, our group reported an immune selection process in a human metastatic melanoma in which early onset of HLA-I loss caused by sequential mutations of the B2M gene led to a gradual decrease in immunogenicity.29, 37, 38 Interestingly, we found no B2M mutations in our DNA analyses of HLA-I negative lung tumors in the present study. In contrast, the RT-PCR experiments revealed a strong down-regulation of B2M and heavy chain gene transcription. We can conclude that lung cancer possesses two independent molecular mechanisms responsible for the HLA-I total loss: HLA haplotype loss, and transcriptional downregulation of HLA-I genes. The possibility that the heavily infiltrated HLA class I positive and TIL-free HLA class I negative tumors are completely different tumor types cannot be ruled out. However, we favor the proposition that, in most cases, the distinct tumor tissue structures and T cell infiltration patterns represent different phases of tumor development. Our hypothesis is based on the observation that SCLC originates from healthy HLA I positive cells. A dynamic interaction between tumor cells and CD8 positive cells is reflected in the observation of heterogeneous tumor samples with different percentages of HLA I positive and negative tumor cells.27 Additionally, we have consistent data pointing to the existence of a chronological sequence in the appearance of tumor HLA class I loss variants in successive metastatic lesions obtained from patients with melanoma and bladder cancer.29, 38, 39 These observations support our hypothesis, based on our ongoing research, that there are two phases of tumor evolution with distinct patterns of immune cell infiltration. In phase 1, when the tumor is HLA-I positive or heterogeneous, it contains lymphocytes and macrophages, and there is an immune-permissive TME in which tumor and infiltrating T cells are in close contact with each other (Fig. 1). In this phase, stroma cannot be distinguished from tumor tissue when staining for HLA-I (Fig. 1). We observed a positive correlation between the presence of tumor infiltrating CD8+ T cells (TIL pattern) and positive tumor HLA-I expression [Supporting Information Fig. S2(a)], and a similar observation has previously been reported in lung cancer.33 In the present study, HLA-I expression was also found to be correlated with the intra- and peritumoral distribution of CD8+ T lymphocytes, which is in turn associated with different tumor evolution phases with distinct tissue reorganization patterns and M1/M2 ratios. In phase 1, it is likely that tumor cells with a \"normal” HLA-I expression are destroyed by CTLs during antigen presentation via the tumor cell surface HLA-β2m-peptide complex. We highlight that all HLA-DR positive tumor tissues (six cases) contained TILs, regardless of their HLA-I status (Table 2). We support the idea that HLA I positive tumor cells are actively killed by CTLs in phase I, leading to a progressive T-cell immune selection of HLA-I deficient tumor cells (phase II). We do not know precisely how long it may take to pass from phase I to phase II, but we could speculate that this period would not be prolonged. Phase II (Fig. 2) is characterized by the absence of tumor infiltrating cells and a clear-cut separation between tumor and stroma cells. T lymphocytes and other leukocytes remain outside the tumor mass, probably because all HLA-I positive tumor cells have been eliminated. We believe that the peripheral distribution of leukocytes in the stroma, outside tumor tissues, is frequent in various types of cancer and is associated with HLA-I loss or downregulation, i.e., an immune escape phenotype (Fig. 2). In this case, tumor escape variants are \"encapsulated” and surrounded by different leukocytes, with a clear barrier between tumor and stroma, in a non-immune-permissive TME (Fig. 2). In this regard, it can be theoretically proposed that NK cells attack HLA-I negative tumors and destroy them, but we found no significant presence of NK cells in the studied tumors. It is difficult to explain the lack of NK cells at the tumor site, but it is possible that the immunosuppressive tumor microenvironment alters NK activity and limits NK cell recruitment from healthy distal lung tissue to the tumor nest.40 We also observed major quantitative changes in the M2/M1 macrophage ratio in phase II, with a predominance of M2 repair-type over M1/inflammatory macrophages. These M2 cells promote wound healing and tissue repair, tumor progression and stroma deposition, and inhibit adaptive immunity through their production of growth factors, cytokines and angiogenic/chemotactic factors.41 In addition, a dense matrix reaction containing numerous FAP+ cancer-associated fibroblasts is also present in HLA-I negative tumor samples (Figs. 4a and 4b). It has been reported that IL-10 and TGF-beta produced by these cells are responsible for HLA-I downregulation, protecting tumor cells from immune recognition by specific CTLs.42 We favor the notion that this stromal reaction contributes to the creation of an immune-privileged compartment around HLA-I negative tumor nests, generating an encapsulated tumor and excluding and limiting the capacity of T-cells to infiltrate and accumulate among tumor cells. We hypothesize that the Phase II pattern is likely the most commonly observed one because of its longer duration during the natural history of tumor development. This may also explain why this tissue structure linked to the absence of HLA-I is frequently observed in the routine pathological diagnosis of tumor tissues. In fact, our group and other researchers observed the same tissue structural organization in various human tumors with HLA-I loss, including HLA-I deficient melanomas, colorectal, breast, bladder and prostate cancers, and other types of malignancy.10, 13, 21 It is noteworthy that this immune escape type of tumor tissue structure (Fig. 2) resembles that observed in different TH2 granulomas, in which the pathogen cannot be destroyed but is isolated from the body by a biological barrier.43 An alternative hypothesis that must be considered is that these are two distinct types of tumor (HLA class I-positive and HLA class I-negative) rather than different development phases, with profoundly different biological characteristics associated with the anti-tumoral efficacy of the local immunity response. It is possible that some HLA-I positive tumors use escape methods other than HLA-loss related mechanisms, in which case the additional loss of tumor HLA would not appear to offer a selective advantage; therefore, the hypothesis of two development phases would not be supported in these cases. Finally, immune checkpoint therapy requires the molecular integrity of HLA-I molecules and only benefits a fraction of patients with different types of cancer, including NSCLC,44, 45 Interestingly, the response rate to pembrolizumab was reported to be 45.2% in one series of NSCLC patients,46 similar to the rate of HLA-I loss mediated by reversible and non-reversible (LOH) mechanisms in the present study. We suggest that HLA class I positive tumors may be more susceptible to treatment with immune checkpoint inhibitors because the mechanism of antigen recognition remains unaltered and the pattern of stromal reactivity and tumor infiltration is \"permissive”. In contrast, HLA-negative tumors (especially those with loss-of-function irreversible truncating mutations in the genes encoding HLA, B2M, APM and IFNG signaling pathway24, 47-49) do not have signal 1 for lymphocyte activation or an adequate infiltration with CD8+ T cells, which are trapped outside the tumor nest in the stroma and have no direct contact with cancer cells (non-permissive pattern). This pattern may be related to the acquired resistance to PD-1 blockade reported in cancer patients. In fact, truncating mutations in the B2M, and JAK1/JAK2 genes have recently been described in recurrent lesions from four patients with metastatic melanoma.50 Given these observations of a stromal rearrangement in HLA-I negative tumors that limits T cell trafficking within the tumor, a combination of checkpoint blockade therapy with regimens targeting stroma and the upregulation of antigen presentation may be beneficial for patients harboring soft HLA-I molecular lesions. The authors thank Eva Ma García and Svetlana Zinchenko for technical assistance and Dr. Natalia Aptsiauri for critical review of the manuscript. This study is part of the doctoral thesis of F. Perea. Additional Supporting Information may be found in the online version of this article. Please note: The publisher is not responsible for the content or functionality of any supporting information supplied by the authors. Any queries (other than missing content) should be directed to the corresponding author for the article. 1Ladanyi A, Somlai B, Gilde K, et al. T-cell activation marker expression on tumor-infiltrating lymphocytes as prognostic factor in cutaneous malignant melanoma. Clin Cancer Res 2004; 10: 521– 30.504. 2Schumacher K, Haensch W, Roefzaad C, et al. Prognostic significance of activated CD8+ T cell infiltration within esophageal carcinomas. Cancer Res 2001; 61: 3932– 36. 3Naito Y, Saito K, Shiiba K, et al. CD8+ T cells infiltrated within cancer cell nests as a prognostic factor in human colorectal cancer. Cancer Res 1998; 58: 3491– 4. 4Galon J, Costes A, Sanchez-Cabo F, et al. Type, density, and location of immune cells within human colorectal tumors predict clinical outcome. Science 2006; 313: 1960– 4. 5Bernal M, Concha A, Sáenz-López P, et al. Leukocyte infiltrate in gastrointestinal adenocarcinomas is strongly associated with tumor microsatellite instability but not with tumor immunogenicity. Cancer Immunol Immunother 2011; 60: 869– 82. 6Ryschich E, Notzel T, Hinz U, et al. Control of T cell mediated immune response by HLA class I in human pancreatic carcinoma. Clin Cancer Res 2005; 11: 498– 504. 7Zhang L, Conejo-Garcia JR, Katsaros D, et al. Intratumoral T cells, recurrence, and survival in epithelial ovarian cancer. N Engl J Med 2003; 348: 203– 13. 8Nakakubo Y, Miyamoto M, Cho Y, et al. Clinical significance of immune cell infiltration within gallbladder cancer. Br J Cancer 2003; 89: 1736– 42. 9Menard S, Tomasic G, Casalini P, et al. Lymphoid infiltration as a prognostic variable for early-onset breast carcinomas. Clin Cancer Res 1997; 3: 817– 9. 10Carretero FJ, Del Campo AB, Flores-Martín JF, et al. Frequent HLA class I alterations in human prostate cancer: molecular mechanisms and clinical relevance. Cancer Immunol Immunother 2016; 65: 47– 59. 11Johnson SK, Kerr KM, Chapman AD, et al. Immune cell infiltrates and prognosis in primary carcinoma of the lung. Lung Cancer 2000; 27: 27– 35. 12Galon J, Mlecnik B, Bindea G, et al. Towards the introduction of the \'Immunoscore\' in the classification of malignant tumours. J Pathol 2014; 232: 199– 209. 13Salmon H, Franciszkiewicz K, Damotte D, et al. Matrix architecture defines the preferential localization and migration of T cells into the stroma of human lung tumors. J Clin Invest 2012; 122: 899– 910. 14Joyce JA, Fearon DT. T cell exclusion, immune privilege, and the tumor microenvironment. Science 2015; 348: 74– 80. 15Garrido F, Cabrera T, Concha A, et al. Natural history of HLA expression during tumour development. Immunol Today 1993; 14: 491– 9. 16Marincola FM, Jafee EM, Hicklin DJ, et al. Escape of human solid tumors from. T cell recognition: molecular mechanisms and functional significance. Adv Immunol 2000; 74: 181– 273. 17Bodmer W, Browning MJ, Krausa P, et al. Tumour escape from immune response by variation in HLA expression and other mechanism. Ann N Y Acad Sci 1993; 690: 42– 49. 18Koopman LA, Corver WE, Van Der Slik AR, et al. Multiple genetic alterations at chromosome 6p cause frequent and heterogeneous HLA class I antigen loss in cervical cancer. J Exp Med 2000; 191: 961– 76. 19Seliger B, Stoehr R, Handke D, et al. Association of HLA class I antigen abnormalities with disease progression and early recurrence in prostate cancer. Cancer Immunol Immunother 2009; 59: 529– 40. 20Challa-Malladi M, Lieu YK, Califano O, et al. Combined genetic inactivation of β2-Microglobulin and CD58 reveals frequent escape from immune recognition in diffuse large B cell lymphoma. Cancer Cell 2011; 20: 728– 40. 21Garrido F, Cabrera T, Lopez-Nevot MA, et al. HLA class I antigens in human tumors. Adv Cancer Res 1995; 67: 155– 95. 22Kloor M, Becker C, Benner A, et al. Immunoselective pressure and human leukocyte antigen class I antigen machinery defects in microsatellite unstable colorectal cancers. Cancer Res 2005; 65: 6418– 24. 23Angell TE, Lechner MG, Jang JK, et al. MHC class I loss is a frequent mechanism of immune escape in papillary thyroid cancer that is reversed by interferon and selumetinib treatment in vitro. Clin Cancer Res 2014; 20: 6034– 44. 24Garrido F, Cabrera T, Aptsiauri N. \" Hard” and \"Soft” lesions underlying the HLA class I alterations in cancer cells: implications for immunotherapy. Int J Cancer 2010; 127: 249– 56. 25Travis WD, Colby TV, Corrin B, eds. Histological typing of lung and pleural tumours, 3rd ed. Heidelberg: Springer, 1999. 156p. 26Sobin L, Gospodarowiaz M, Wittekind CH. TNM classification of malignant tumours UICC 2009, 7th edn. Chichester: Wiley, 2010. 310p. 27Garrido F, Cabrera T, Accolla RS, et al. HLA and cancer: 12th International Histocompatibility Workshop study. In: D Charron, ed. Genetic diversity of HLA. Functional and medical implication. Proc. Twelfth International Histocompatibility Workshop and Conference, Vol. II. France: EDK, Sevres; 1997. 445– 52. 28Vogelstein B, Papadopoulos N, Velculescu VE, et al. Cancer genome landscapes. Science 2013; 339: 1546– 58. 29del Campo AB, Kyte JA, Carretero J, et al. Immune escape of cancer cells with beta2-microglobulin loss over the course of metastatic melanoma. Int J Cancer 2014; 134: 102– 13. 30Garrido F, Ruiz-Cabello F, Cabrera T, et al. Implications for immunosurveillance of altered HLA class I phenotypes in human tumours. Immunol Today 1997; 18: 89– 95. 31Redondo M, Ruiz-Cabello F, Concha A, et al. Altered HLA class I expression in non-small cell lung cancer is independent of c-myc activation. Cancer Res 1991; 51: 2463– 8. 32Redondo M, Concha A, Oldiviela R, et al. Expression of HLA class I and II antigens in bronchogenic carcinomas: its relationship to cellular DNA content and clinical-pathological parameters. Cancer Res 1991; 51: 4948– 54. 33Kikuchi E, Yamazaki K, Torigoe T, et al. HLA class I antigen expression is associated with a favorable prognosis in early stage non-small cell lung cancer. Cancer Sci 2007; 98: 1424– 30. 34Baba T, Shiota H, Kuroda K. Clinical significance of human leukocyte antigen loss and melanoma-associated antigen 4 expression in smokers of non-small cell lung cancer patients. Int J Clin Oncol 2013; 18: 997– 1004. 35So T, Takenoyama M, Mizukami M, et al. Haplotype loss of HLA class I antigen as an escape mechanism from immune attack in lung cancer. Cancer Res 2005; 65: 5945– 52. 36Maleno I, Cabrera CM, Cabrera T, et al. Distribution of HLA class I altered phenotypes in colorectal carcinomas: high frequency of HLA haplotype loss associated with loss of heterozygosity in chromosome region 6p21. Immunogenetics 2004; 56: 244– 53. 37Bernal M, Ruiz-Cabello F, Concha A, et al. Implication of the β2-microglobulin gene in the generation of tumor escape phenotypes. Cancer Immunol Immunother 2012; 61: 1359– 71. 38Paschen A, Méndez RM, Jimenez P, et al. Complete loss of HLA class I antigen expression on melanoma cells: a result of successive mutational events. Int J Cancer 2003; 103: 759– 67. 39Carretero R, Cabrera T, Gil H, et al. Bacillus Calmette-Guerin immunotherapy of bladder cancer induces selection of human leukocyte antigen class I-deficient tumor cells. Int J Cancer 2011; 129: 839. 40Valenzuela-Membrives M, Perea-García F, Sanchez-Palencia A, et al. Progressive changes in composition of lymphocytes in lung tissues from patients with non-small-cell lung cancer. Oncotarget, 2016; doi:10.18632/oncotarget.12264. 41Mills CD, Lenz LL, Harris RA. A breakthrough: macrophage-directed cancer immunotherapy. Cancer Res 2016; 76: 513– 6. 42Chen XH, Liu ZC, Zhang G, et al. TGF-β and EGF induced HLA-I downregulation is associated with epithelial-mesenchymal transition (EMT) through upregulation of snail in prostate cancer cells. Mol Immunol 2015; 65: 34– 42. 43Mukhopadhyay S, Farver CF, Vaszar LT, et al. Causes of pulmonary granulomas: a retrospective study of 500 cases from seven countries. J Clin Pathol 2012; 65: 51– 57. 44Spranger S, Koblish HK, Horton B, et al. Mechanism of tumor rejection with doublets of CTLA-4, PD-1/PD-L1, or IDO blockade involves restored IL-2 production and proliferation of CD8(+) T cells directly within the tumor microenvironment. J Immunother Cancer 2014; 2: 3. 45Herbst RS, Baas P, Kim DW, et al. Pembrolizumab versus docetaxel for previously treated, PD-L1-positive, advanced non-small-cell lung cancer (KEYNOTE-010): a randomised controlled trial. Lancet 2015; pii:. S0140-6736(15)01281–7. 46Garon EB, Rizvi NA, Hui R, et al. Pembrolizumab for the treatment of non-small-cell lung cancer. N Engl J Med 2015; 372: 2018– 28. 47Aptsiauri N, Carretero R, Garcia-Lora A, et al. Regressing and progressing metastatic lesions: resistance to immunotherapy is predetermined by irreversible HLA class I antigen alterations. Cancer Immunol Immunother 2008; 57: 1727– 33. 48Garrido F, Aptsiauri N, Doorduijn EM, et al. The urgent need to recover MHC class I in cancers for effective immunotherapy. Curr Opin Immunol 2016; 39: 44– 51. 49Seliger B, Ruiz-Cabello F, Garrido F. IFN inducibility of major histocompatibility antigens in tumors. Adv Cancer Res 2008; 101: 249– 76. 50Zaretsky JM, Garcia-Diaz A, Shin DS, et al. Mutations associated with acquired resistance to PD-1 blockade in melanoma. N Engl J Med 2016; 375: 819– 29. The full text of this article hosted at iucr.org is unavailable due to technical difficulties. Please check your email for instructions on resetting your password. If you do not receive an email within 10 minutes, your email address may not be registered, and you may need to create a new Wiley Online Library account. Can\'t sign in? Forgot your username? Enter your email address below and we will send you your username If the address matches an existing account you will receive an email with instructions to retrieve your username
本文链接: https://www.ebiomall.cn/b67-bd/info-15254.html
免责声明 本文仅代表作者个人观点,与本网无关。其创作性以及文中陈述文字和内容未经本站证实,对本文以及其中全部或者部分内容、文字的真实性、完整性、及时性本站不做任何保证或承诺,请读者仅作参考,并请自行核实相关内容。
版权声明 未经蚂蚁淘授权不得转载、摘编或利用其他方式使用上述作品。已经经本网授权使用作品的,应该授权范围内使用,并注明“来源:蚂蚁淘”。违反上述声明者,本网将追究其相关法律责任。
相关文章
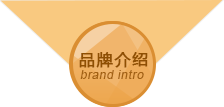
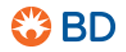
▍
品牌分类
▍
官网分类