新闻动态
RH5–Basigin interaction plays a major role in the host...
2025-05-07
Contributed by Beatrice H. Hahn, November 5, 2013 (sent for review September 20, 2013) SignificancePlasmodium falciparum is responsible for almost all malaria-related deaths and belongs to a family of parasites that infect African apes. In their native habitat, these parasites exhibit strict host tropism, with human P. falciparum having never been found in wild-living chimpanzees and gorillas. Our research provides a molecular explanation for this phenomenon by showing that the interaction between a parasite protein (RH5) and its erythrocyte cell surface receptor (Basigin) is species specific and mirrors the observed host–parasite tropism. Our findings also reveal how a parasite responsible for one of the world’s major health problems has evolved its relationship with the human host and identify specific changes in the Basigin receptor that could make human erythrocytes resistant to infection by P. falciparum.AbstractPlasmodium falciparum, the cause of almost all human malaria mortality, is a member of the Laverania subgenus which infects African great apes. Interestingly, Laverania parasites exhibit strict host specificity in their natural environment: P. reichenowi, P. billcollinsi, and P. gaboni infect only chimpanzees; P. praefalciparum, P. blacklocki, and P. adleri are restricted to gorillas, and P. falciparum is pandemic in humans. The molecular mechanism(s) responsible for these host restrictions are not understood, although the interaction between the parasite blood-stage invasion ligand EBA175 and the host erythrocyte receptor Glycophorin-A (GYPA) has been implicated previously. We reexamined the role of the EBA175–GYPA interaction in host tropism using recombinant proteins and biophysical assays and found that EBA175 orthologs from the chimpanzee-restricted parasites P. reichenowi and P. billcollinsi both bound to human GYPA with affinities similar to that of P. falciparum, suggesting that the EBA175–GYPA interaction is unlikely to be the sole determinant of Laverania host specificity. We next investigated the contribution of the recently discovered Reticulocyte-binding protein Homolog 5 (RH5)–Basigin (BSG) interaction in host-species selectivity and found that P. falciparum RH5 bound chimpanzee BSG with a significantly lower affinity than human BSG and did not bind gorilla BSG, mirroring the known host tropism of P. falciparum. Using site-directed mutagenesis, we identified residues in BSG that are responsible for the species specificity of PfRH5 binding. Consistent with the essential role of the PfRH5–BSG interaction in erythrocyte invasion, we conclude that species-specific differences in the BSG receptor provide a molecular explanation for the restriction of P. falciparum to its human host.surface plasmon resonanceprotein interactionsThe most deadly of the malaria parasites, Plasmodium falciparum, is highly divergent from the other species of Plasmodium known to infect humans (1⇓–3), with its closest relatives comprising a group of chimpanzee and gorilla parasites from the subgenus Laverania (3⇓⇓⇓–7). Despite the origin of P. falciparum as a zoonosis, and the continuing coexistence of humans and apes in West and Central Africa, extensive field studies have failed to detect P. falciparum in wild-living chimpanzees and gorillas (5). Although there are reports of P. falciparum infecting chimpanzees either in certain captive settings (2) or following splenectomy and deliberate transfer of P. falciparum-infected human blood (8⇓–10), the resulting infections have low parasitemia and are not known to result in malignant tertian malaria, suggesting a host-specific barrier for replete infection. The existence of host-specific barriers within the Laverania subgenus is supported further by the strict host specificity exhibited by ape Laverania parasites in the wild: Plasmodium reichenowi, Plasmodium billcollinsi, and Plasmodium gaboni infect only chimpanzees, and Plasmodium praefalciparum, Plasmodium blacklocki, and Plasmodium adleri are restricted to gorillas (3⇓⇓–6).The molecular basis for the host tropism of P. falciparum and other Laverania parasites is not currently understood and is difficult to investigate experimentally, because no ape Laverania parasites have been adapted to in vitro culture, and because ethical considerations clearly preclude the use of endangered African apes for in vivo experiments (3). In principle, the species specificity of known host–parasite interactions could be examined using recombinant proteins, but the technical challenges associated with expressing functional Plasmodium proteins in a recombinant form have made this approach difficult thus far (11). There also are multiple points in the complex Plasmodium life cycle that could represent restriction points, and some evidence suggests that transmission of Laverania to anthropophilic Anopheles species, for example, may be restricted (12). Although several restriction points are possible, the blood stage of Plasmodium infection, which is initiated when host erythrocytes are invaded by the parasite, is of particular interest. Erythrocyte invasion is an obligate stage in the parasite life cycle (13), and the inability of P. falciparum to produce infections of high parasitemia in chimpanzees by transfer of infected blood, despite contrived permissive experimental conditions, suggests that a host-specific barrier for infection exists at the blood stage. Moreover, erythrocyte invasion involves extracellular interactions between several different receptor–ligand pairs which are coevolving rapidly, driven by strong immune selection pressure and functional constraints (14, 15). This rapid coevolution means that interactions between parasite blood-stage ligands and erythrocyte receptors quickly could become host-specific, isolating a certain parasite species within a single host. Given these findings, erythrocyte invasion therefore is likely to represent a significant restriction point in determining host tropism.P. falciparum erythrocyte invasion depends on a partially redundant set of parasite ligands and erythrocyte receptors (14, 16⇓–18), and there is experimental support for the suggestion that at least two of these interactions may be involved in P. falciparum host tropism. The interaction between Plasmodium falciparum Erythrocyte Binding Antigen-175 (PfEBA175) and the erythrocyte receptor Glycophorin-A (GYPA) is important for invasion and is known to require sialic acid residues displayed on GYPA (19, 20). The sialic acid content of human GYPA differs from that of other apes because humans lack a functional cytidine monophosphate-N-acetylneuraminic acid hydroxylase (CMAH) enzyme. Consequently, human GYPA contains only N-Acetylneuraminic acid (Neu5Ac) sialic acids, whereas chimpanzees and gorillas, both of which have an active CMAH gene, contain a mixture of both Neu5Ac and its hydroxylated derivative, N-Glycolylneuraminic acid (Neu5Gc), with Neu5Gc being more abundant (21). This difference in GYPA sialic acid content led Martin et al. (10) to propose that the restriction of P. falciparum to humans results from the sialic acid-binding specificity in the PfEBA175–GYPA interaction. The cocrystal structure of the glycan-binding regions of P. falciparum EBA175 in complex with a Neu5Ac-containing glycan derivative (22) followed by modeling of the equivalent EBA175 sequence from the chimpanzee-restricted parasite, P. reichenowi (23), suggested a structural basis for this binding specificity. However, this hypothesis is inconsistent with a much earlier study by Orlandi et al. (24) in which the binding of native PfEBA175 to human erythrocytes was observed to be potently inhibited by soluble Neu5Gc and oligosaccharides containing Neu5Acα2–3Gal but not by Neu5Ac as a monosaccharide. This glycan-binding specificity was confirmed in a recent biochemical investigation using a recombinant protein consisting of the entire ectodomain of PfEBA175 (25). Furthermore, the sialic acid specificity of PfEBA175 orthologs cannot explain the restriction of ape Laverania parasites to their respective chimpanzee and gorilla hosts, both of which carry a functional CMAH gene (5). Therefore there is reasonable doubt about the importance of the EBA175–GYPA interaction in Laverania host tropism. A second and unrelated invasion ligand, Plasmodium falciparum Reticulocyte-binding protein homolog 5 (PfRH5) has also been implicated in host tropism by governing the ability of P. falciparum to infect New World Aotus monkeys (26, 27). The erythrocyte cell-surface receptor of PfRH5, Basigin (BSG), was identified only recently (28), and so the contribution of the PfRH5–BSG interaction toward determining host-species selectivity in P. falciparum is unknown.We recently have developed technology that enables the recombinant expression of P. falciparum cell-surface and secreted proteins in a functional form using a mammalian expression system (29). We have used this method to identify novel host–parasite interactions (28, 30), but here we apply it to investigate the relative roles of the EBA175–GYPA and PfRH5–BSG interactions as determinants of P. falciparum host tropism.ResultsEBA175 RII Orthologs from the Chimpanzee-Restricted Parasites P. billcollinsi and P. reichenowi Bind Native Human GYPA.To investigate the molecular determinant(s) underlying the species tropism of P. falciparum, we first asked whether EBA175 homologs were present in other Laverania parasites by screening fecal DNA from Laverania-infected wild-living African apes for EBA175 sequences. Because of the lower copy numbers of nuclear Plasmodium genomes as compared with mitochondrial DNA, it is technically challenging to amplify large nuclear gene fragments from fecal DNA. Nonetheless, we were able to amplify thirty-seven 397-bp fragments spanning the receptor-binding region (RII) of EBA175 from 22 chimpanzee and gorilla samples (Table S1). Phylogenetic analysis of the corresponding sequences identified three chimpanzee- and three gorilla-specific clades, thus identifying EBA175 orthologs in each of the six ape Laverania species (Fig. S1). Because all Laverania species seemed to encode an EBA175 homolog, it could play a role in host tropism across the subgenus. To test this hypothesis, we attempted to amplify an entire RII gene fragment for functional binding studies. Although representatives of all ape Laverania species were included, we were able to amplify the complete 1.9-kb coding region of EBA175 RII from only one chimpanzee fecal sample (KApts1680), presumably because of the fragmented nature of fecal DNA samples. Amplification was carried out using single-genome amplification (SGA) followed by direct amplicon sequencing because this approach eliminates Taq polymerase-induced recombination and nucleotide-substitution errors in finished sequences and provides a proportional representation of parasite sequences that are present in vivo (5). The newly derived EBA175 RII sequence fell within a cluster tentatively classified as P. billcollinsi (Fig. S1). An alignment of its deduced amino acid sequence revealed a high degree of similarity ( 80%) with the orthologous protein domains in P. falciparum and P. reichenowi.If the interaction between EBA175 and GYPA was a primary determinant of Laverania parasite host tropism, we reasoned that the GYPA and EBA175 orthologs from different host and Laverania species should be species specific and match the known host–parasite pairings. To test this hypothesis, we constructed expression vectors encoding P. billcollinsi, P. reichenowi, and P. falciparum EBA175 RII sequences and expressed them in mammalian cells. The resulting proteins were of the expected size and were properly folded, because they were recognized by anti-EBA175 monoclonal antibodies specific for heat-labile epitopes in the tandem Duffy binding-like domains (Fig. S2). To determine their binding specificities, we coated fluorescent microbeads with the RII proteins and used flow cytometry to quantify their ability to bind human erythrocytes. The results revealed robust binding of the chimpanzee-restricted P. billcollinsi and P. reichenowi EBA175 RII orthologs to human erythrocytes, as well as the P. falciparum positive control (Fig. 1A). Binding of the EBA175-coated beads was abolished by treatment of erythrocytes with Vibrio cholera neuraminidase to remove sialic acid residues, as is consistent with the requirement for Neu5Acα-2,3-Gal glycan moieties for EBA175 binding (Fig. 1A). To determine whether the EBA175 orthologs bound native GYPA directly, we screened them against GYPA extracted from human erythrocytes using a modified version of the avidity-based extracellular interaction screening (AVEXIS) assay, an approach designed to detect transient extracellular protein interactions (28, 30, 31). Again, both P. billcollinsi and P. reichenowi EBA175 RII orthologs bound native human GYPA in a sialic acid-dependent manner (Fig. 1B). Finally, we directly measured the binding affinities of EBA175 RII from both P. billcollinsi and P. reichenowi for native human GYPA using surface plasmon resonance (SPR) and compared it with P. falciparum. Serial dilutions of the EBA175 proteins from the three Plasmodium species were injected over GYPA immobilized on a sensor chip, and the equilibrium dissociation constants (KD) for the interactions were calculated by fitting each dataset to a steady-state binding model. The KD values measured for both P. billcollinsi and P. reichenowi EBA175 RIIs were all within the same order of magnitude as those for P. falciparum (Fig. 1C). Taken together, these data suggest that the EBA175–GYPA interaction is not solely responsible for the differential host tropism of P. falciparum and ape Laverania parasites.Download figureOpen in new tabDownload powerpointFig. 1. Human GYPA binds EBA175 RII orthologs from P. falciparum, P. reichenowi, and P. billcollinsi with similar affinities. In each panel, the data corresponding to the EBA175 RII proteins from each species are color-coded: blue, P. reichenowi; green, P. billcollinsi; and red, P. falciparum (positive control). (A) Fluorescent beads coated with the EBA175 RII orthologs from chimpanzee-restricted parasites P. reichenowi and P. billcollinsi bind human erythrocytes in a sialic acid-dependent manner. Solid colored lines, untreated erythrocytes; dotted colored lines, neuraminidase-treated erythrocytes; black line, negative control (Cd4-coated beads). A representative experiment is shown. (B) The EBA175 RII orthologs from P. reichenowi and P. billcollinsi bind native human GYPA in a sialic acid-dependent manner using the AVEXIS assay. Native, sialylated (Left) and neuraminidase-treated, asialyl (Right) GYPA was biotinylated and immobilized on a streptavidin-coated plate. Binding of the EBA175 RII orthologs was assessed using β-lactamase–tagged pentamers using the AVEXIS assay. Data are shown as mean ± SD; n = 3. (C) Binding affinities at equilibrium of the EBA175 RII orthologs for native human GYPA were within the same order of magnitude, as determined by SPR. The reference-subtracted binding data obtained by injecting twofold dilutions of the purified EBA175 RII proteins at 20 µL/min over biotinylated GYPA immobilized on a streptavidin-coated sensor chip were plotted as a binding curve once equilibrium was reached, and the KD was calculated by globally fitting a steady-state 1:1 interaction model. KD is shown as mean ± SEM. Biotinylated β-d-glucose was used as the reference.Gorilla BSG Does Not Bind PfRH5, and Chimpanzee BSG Binds with Much Reduced Affinity Compared with Human BSG.The lack of species-specific EBA175-GYPA binding prompted us to search for other interactions that might explain the human-specific tropism of P. falciparum. Recently, the Ok blood group antigen BSG has been shown to be the erythrocyte receptor for P. falciparum RH5, a secreted blood-stage protein that belongs to a paralogous gene family called the \"reticulocyte-binding protein homologs” (RHs) (28). Importantly, and in contrast to other known P. falciparum invasion ligand–erythrocyte receptor interactions, including EBA175–GYPA, RH5–BSG binding was found to be both essential and universally required for erythrocyte invasion by the parasite (28). To investigate the role of the PfRH5–BSG interaction in P. falciparum host tropism, we expressed the entire ectodomains of the BSG orthologs from human, chimpanzee (Pan troglodytes), and gorilla (Gorilla gorilla) as soluble recombinant proteins (Fig. S3). Western blotting, size-exclusion chromatography, and ELISA-based immunoreactivity assays were used to demonstrate that the recombinant BSG proteins were expressed at the expected sizes and showed evidence of correct folding (Fig. S4). We then systematically tested the three ape BSG orthologs for their ability to interact with P. falciparum RH5 using AVEXIS. Using this approach, we found that PfRH5 clearly bound human BSG, as expected, but bound the chimpanzee and gorilla orthologs either poorly or not at all (Fig. 2A). We quantified the differences in binding strength (Fig. 2B) and showed that PfRH5 bound human BSG with more than 10-fold greater affinity compared with chimpanzee BSG (KDs = 0.8 µM for human and 10.4 µM for chimpanzee). The reduced binding affinity of chimpanzee BSG for PfRH5 was caused by a twofold slower association and sixfold faster dissociation in complex formation, relative to the human BSG–PfRH5 interaction (Fig. 2B). We did not detect any binding of PfRH5 to gorilla BSG despite the sensitivity of the SPR technique, which, under the conditions used, should have detected interactions as weak as 50 µM or lower (Fig. S5). These findings are consistent with chimpanzees being susceptible to infection with human P. falciparum under certain circumstances, whereas P. falciparum never has been detected in gorillas, either in captivity or in the wild. Thus, our cross-species binding data of PfRH5 with the ape BSG orthologs perfectly matched the known host tropism of P. falciparum.Download figureOpen in new tabDownload powerpointFig. 2. Interactions between PfRH5 and primate BSG orthologs are species specific. (A) Binding of PfRH5 to human (HsBSG), chimpanzee (PtBSG), and gorilla (GgBSG) BSG. Biotinylated PfRH5 (bait) was immobilized on streptavidin-coated plates and probed with pentameric, β-lactamase–tagged (prey) forms of the indicated BSG orthologs. Biotinylated Cd4 was used as the negative control bait, and β-lactamase–tagged Cd4 was used as the negative control prey. (B) Kinetic analysis of the human (Upper) and chimpanzee (Lower) PfRH5–BSG interaction. Twofold serial dilutions of PfRH5 were injected at 100 µL/min over the indicated BSG orthologs immobilized in different flow cells of a streptavidin-coated sensor chip. The association and dissociation rate constants (ka and kd) were calculated by nonlinear curve fitting to the reference-subtracted sensorgrams. The equilibrium dissociation constant (KD) was calculated from kd/ka and the half-life (t1/2) from ln2/kd. HsBSG-PfRH5: ka = (3.0 ± 0.7) × 105⋅M−1⋅s−1, kd = 0.246 ± 0.002⋅s−1; PtBSG-PfRH5: ka = (1.40 ± 0.02) × 105⋅M−1⋅s−1, kd = 1.45 ± 0.08⋅s−1. The ka and kd values are means ± SEM from two independent experiments.Identification of BSG Residues That Contribute to the Species-Specific Recognition by PfRH5.An alignment of the human, gorilla, and chimpanzee BSG protein sequences revealed a high level of conservation, suggesting that differential recognition by PfRH5 must depend on only a small number of residues (Fig. S3). Because chimpanzee BSG exhibited some PfRH5 binding, we first attempted to increase its affinity by introducing the equivalent human residues using site-directed mutagenesis. Chimpanzee and human BSG are identical in their first Ig-like domain, and so we reasoned that the reduced affinity of chimpanzee BSG for PfRH5 was caused by one or more of the nine polymorphic positions in the second, membrane proximal, Ig-like domain. All nine mutants were expressed and shown to fold correctly using a panel of anti-BSG monoclonal antibodies, which also revealed that the epitope of the invasion-blocking anti-BSG antibody MEM-M6/6 included the lysine at position 191 (Fig. S6). All mutants then were tested by AVEXIS for their ability to interact with PfRH5. Strikingly, only the E191K mutant appeared to have gained the ability to interact with PfRH5 (Fig. 3A). Subsequent analysis by SPR confirmed this observation; indeed, surprisingly, the chimpanzee BSG E191K mutant was able to bind PfRH5 with 2.5-fold higher affinity than human BSG (Fig. 3B). The lysine residue at position 191 of human BSG therefore appears to play an important role in the interaction with PfRH5, and the presence of an oppositely charged glutamic acid at the corresponding position in chimpanzee BSG likely is responsible for its much lower affinity for the P. falciparum ligand. This residue, however, is not sufficient for PfRH5 binding, because gorilla BSG, like human BSG, contains a lysine at position 191 (Fig. S3). Moreover, it previously has been established that both Ig-like domains of human BSG are necessary for PfRH5 binding (28). Because no binding of PfRH5 to gorilla BSG was detectable, it seemed likely that more than one of the polymorphic sites between the gorilla and human protein contributed to the loss of PfRH5 binding, including those in the membrane-distal domain 1. We thus mutated all residues in the first Ig-like domain of human BSG to those present at the corresponding positions in the gorilla sequence, including an insertion of a histidine residue at position 103. Again, all mutants were expressed and shown to be correctly folded using the panel of anti-BSG monoclonal antibodies (Fig. S6). Three of the five mutants, human BSG F27L, Q100K, and +H103, showed significantly reduced binding to PfRH5 relative to human BSG (Fig. 3C). We confirmed and quantified these findings using SPR, which revealed the affinities of the mutants F27L, Q100K, and +H103 for PfRH5 were lower than that of human BSG by approximately eightfold,11-fold, and 10-fold, respectively (Fig. 3D). The inability of gorilla BSG to bind PfRH5 therefore is likely to be caused by differences at multiple positions, including residues at positions 27, 100, and 103. Finally, mapping these residues onto the known structure of human BSG (32) revealed that their R-groups were solvent exposed, as is consistent with their role in mediating protein interactions (Fig. 3E). The spatial location of these residues suggests that PfRH5 interaction is likely to occur across the glycan-free surface on one side of the BSG molecule encompassing both Ig-like domains and would require stabilization of the rotation of both domains relative to one another, as observed in the BSG solution structure (Fig. 3E) (33).Download figureOpen in new tabDownload powerpointFig. 3. Identification of BSG residues that contribute to the species-specific recognition of PfRH5. (A) Chimpanzee BSG (PtBSG) and a panel of humanized mutants are presented as biotinylated baits and probed for binding with a β-lactamase–tagged PfRH5 pentamer prey using the AVEXIS assay. Data are shown as mean ± SD; n = 2. (B) Dissociation-phase SPR analysis of PfRH5 from human BSG (HsBSG, black squares), chimpanzee BSG (PtBSG, black triangles), and the PtBSG mutant E191K (red circles). PtBSG (E191K) bound PfRH5 with ka = (2.8 ± 0.6) × 105⋅M−1⋅s−1 and kd = 0.089 ± 0.002⋅s−1; results shown are mean ± SEM from two independent experiments. Data points are reference-subtracted dissociation phases averaged from three different concentrations of PfRH5. One representative experiment is shown (mean ± SD; n = 3). Only every other data point is displayed for ease of comparison. (C) Human BSG (HsBSG) and a panel of variants in which the equivalent residues from gorilla BSG were mutated in HsBSG were presented as baits and tested for binding with a PfRH5 prey using the AVEXIS assay. Data are shown as mean ± SD; n = 2. (D) The binding of human BSG (HsBSG, dark blue line) and three of its site-directed mutants (F27L, light blue; Q100K, purple; and +H103, green) to PfRH5 was analyzed by SPR. Measured parameters were HsBSG (F27L)-PfRH5: ka = (1.4 ± 0.5) × 105⋅M−1⋅s−1, kd = 0.88 ± 0.01⋅s−1; HsBSG (Q100K)-PfRH5: ka = (2.19 ± 0.08) × 105⋅M−1⋅s−1, kd = 2.0 ± 0.4⋅s−1; HsBSG (+H103)-PfRH5: ka = (1.60 ± 0.03) × 105⋅M−1⋅s−1, kd = 1.30 ± 0.09⋅s−1. For ease of comparison, only a single injected concentration of PfRH5 is shown. All values are mean ± SEM from two independent experiments. (E) X-ray crystal structure of human BSG (Protein Data Bank ID: 3B5H). The schematic shows the spatial organization of residues identified as important for species-specific discrimination of PfRH5 binding. The approximate location of the additional histidine (H103) in gorilla BSG is indicated by an arrow.DiscussionPrevious research on the molecular determinants of P. falciparum host tropism had focused on the EBA175–GYPA interaction, which, because of the predominance of the Neu5Gc form of sialic acid in chimpanzees and its absence in humans, provided an explanation for the restriction of P. falciparum to humans and P. reichenowi to chimpanzees (10, 21). However, the nonessential role of EBA175 for the invasion of some P. falciparum strains (19), together with the recent discovery that ape Laverania parasites exhibit strict host tropism despite active CMAH genes in both chimpanzees and gorillas (5), prompted us to reexamine the role of the EBA175–GYPA in host restriction. Although we identified EBA175 homologs in all Laverania species, the fact that EBA175 RII orthologs from chimpanzee-restricted parasites P. reichenowi and P. billcollinsi were able to bind human erythrocytes in a sialic acid-dependent manner and could bind human GYPA with similar affinities as P. falciparum EBA175 RII, suggested that the interaction of EBA175 with GYPA is unlikely to be the sole determinant of host restriction of P. falciparum and ape Laverania parasites more generally. Our results thus differ from previous findings by Martin et al. (10), who reported a much greater binding differential of P. falciparum and P. reichenowi EBA175 RII to human GYPA. It is important to note that the assays used to measure EBA175–GYPA interactions differ significantly in the two studies. The biophysical measurements reported here are based on interactions between purified soluble recombinant EBA175 proteins and purified glycophorin assayed under flow conditions, whereas the previous data relied on static assays in which RII fragments of EBA175 were expressed in a heterologous context on the surface of COS cells and were exposed to whole human or chimpanzee erythrocytes. By using SPR, we have been able to address the host specificity of EBA175–GYPA interactions in a quantitative and directly comparable manner by measuring binding affinities. The proposed lack of PfEBA175 binding to Neu5Gc, which forms the basis of the EBA175-related host restriction hypothesis, also is at odds with the observation that soluble Neu5Gc as a monosaccharide inhibits the binding of native PfEB175 to human erythrocytes with a level of potency similar to that of oligosaccharides containing Neu5Acα-2,3-Gal (24) and our recent finding that a functionally active recombinant protein containing the full-length ectodomain of PfEBA175 binds Neu5Gc as well as Neu5Acα-2,3-Gal (25). We attempted to investigate the specificity of the EBA175–GYPA interaction from the perspective of the host receptor, but we were unable to express human GYPA in a functionally active, recombinant form using our mammalian expression system (25). Based on the available evidence, we therefore propose that the EBA175–GYPA interaction is unlikely to be the sole determinant of host tropism within Laverania parasites, including P. falciparum, but additional experiments using either native or biochemically active recombinant ape GYPA are required to dissect fully the host specificity of the EBA175–GYPA interaction.In contrast, we show here that PfRH5 binding was highly host specific. Recombinant PfRH5 did not interact with gorilla BSG, even using techniques that are sensitive enough to detect very weak binding (KDs ≥50 µM), and bound chimpanzee BSG with a much lower affinity than human BSG. Because the interaction of PfRH5 with human BSG is known to be essential for the invasion of human erythrocytes by P. falciparum (28), it is likely that the loss or greatly reduced affinity of PfRH5 for binding the ape BSG orthologs represents a significant barrier to infection of chimpanzees and gorillas by P. falciparum. It also is known that a lower affinity of the PfRH5–BSG interaction can adversely affect P. falciparum erythrocyte invasion, because Oka− blood group erythrocytes, which carry a homozygous BSG polymorphism that binds PfRH5 with a twofold-lower affinity, are not invaded as efficiently as Oka+ erythrocytes (28). Finally, these findings concur with the identification of PfRH5 variants that are responsible for the species selectivity of P. falciparum in both owl monkeys (Aotus nancymaae) and rats (26, 27). Thus, several lines of evidence suggest that the PfRH5–BSG interaction is a major determinant of host species tropism.Using site-directed mutagenesis, we were able to identify residues that contribute to the host-specific binding of PfRH5 in both chimpanzees and gorillas. As is consistent with the previous observation that both Ig-like domains 1 and 2 of human BSG are required for recognition of PfRH5 (28), the residues we identified are located in both Ig-like domains of human BSG. Interestingly, a single amino acid polymorphism [lysine (K) in human to glutamic acid (E) in chimpanzee] that resulted in a charge switch at position 191 was sufficient to confer PfRH5 binding to chimpanzee BSG. Among the currently available primate BSG sequences, only humans and gorillas encode a lysine at position 191; other primates including orangutan, rhesus macaque, baboon, and marmoset all contain glutamic acid. It will be interesting to see whether Aotus monkeys contain a lysine at the equivalent position in their BSG ortholog once sequences are available. We were able to show that this residue also is part of the epitope for the MEM-M6/6 monoclonal antibody, which is a potent antagonist of the PfRH5–human BSG interaction and erythrocyte invasion (28), and thus its epitope is likely to be located either at or near the PfRH5 interaction surface of human BSG. The discovery of a point mutation in BSG that could preserve BSG function but prevent PfRH5 binding to make erythrocytes refractory to invasion potentially could be exploited therapeutically.In summary, we show here that species-specific differences in the interaction of PfRH5 with ape BSG orthologs provide a plausible explanation for the absence of P. falciparum from wild ape populations. Studies of additional Laverania RH5 proteins will be necessary to elucidate the full extent of the RH5–BSG interaction and its importance for host restriction across the Laverania subgenus and may help us understand how P. falciparum evolved its relationship with its human host.Materials and MethodsFull details are available in SI Materials and Methods.Amplification of Laverania EBA175 Gene Fragments.Laverania EBA175 sequences were amplified from ape fecal samples using single-genome amplification essentially as described (5). GenBank accession numbers for all sequences are listed in Table S1. A larger 1.9-kb fragment corresponding to the entire EBA175 RII (KApts1680_SGA5.9) clustered within the C3 clade (denoted by an asterisk in Fig. S1) and thus was tentatively classified as P. billcollinsi.Protein Production, Purification, and Analysis.P. falciparum and P. reichenowi EBA175 RII and human, gorilla, and chimpanzee BSG sequences were obtained from publicly accessible databases, and expression constructs were chemically synthesized. GYPA preparations were purchased (catalogue nos. G7903 and A9791; Sigma) and biotinylated in vitro using EZ-link sulfo-NHS-biotin (Thermo Scientific). Biotinylated bait and pentameric β-lactamase–tagged prey proteins were produced in HEK293E cells, purified, and gel-filtered as described (28, 30, 34). Western blotting and ELISAs were performed as described (28). Monoclonal antibodies were kind gifts from David Narum of the National Institute of Allergy and Infectious Diseases, National Institutes of Health (Bethesda) (R217 and R218) (35) and Vaclav Horejsi of the Institute of Molecular Genetics (Prague) (MEM-M6 series) (36).Protein Interaction Assays.AVEXIS assays were performed essentially as described (30, 37). Bead-based erythrocyte-binding assays were performed as described (38) using streptavidin-coated Nile red fluorescent 0.4- to 0.6-µm microbeads (Spherotech Inc). Erythrocytes were pretreated with 0.1 mU per 106 cells of Vibrio cholera neuraminidase (Sigma) for 1 h at 37 °C where appropriate. SPR studies were performed as described (28) using a regeneration solution of 5 M NaCl. Equilibrium and kinetic constants were calculated by nonlinear regression fitting to reference-subtracted sensorgrams as described (28).AcknowledgmentsWe thank Cécile Crosnier, Zenon Zenonos, David Narum, and Vaclav Horejsi for important reagents; Gerald Learn for phylogenetic analysis; and Josefin Bartholdson for helpful discussions. This work was supported by the Wellcome Trust Grant 098051, Medical Research Council Grant MR/J002283/1, the University of Pennsylvania Center for AIDS Research Single Genome Amplification Core Core Facility, and National Institutes of Health Grants R37 AI050529, R01 AI58715, and P30 AI045008.Footnotes↵1To whom correspondence may be addressed. E-mail: gw2{at}sanger.ac.uk, jr9{at}sanger.ac.uk, or bhahn{at}upenn.edu.Author contributions: M.W., J.C.R., and G.J.W. designed research; M.W. and W.L. performed research; M.W., B.H.H., J.C.R., and G.J.W. analyzed data; and M.W., B.H.H., J.C.R., and G.J.W. wrote the paper.The authors declare no conflict of interest.This article contains supporting information online at www.pnas.org/lookup/suppl/doi:10.1073/pnas.1320771110/-/DCSupplemental.Freely available online through the PNAS open access option. References↵Bray RS (1958) Studies on malaria in chimpanzees. VI. Laverania falciparum. Am J Trop Med Hyg 7(1):20–24.OpenUrlAbstract/FREE Full Text↵Duval L, et al. (2010) African apes as reservoirs of Plasmodium falciparum and the origin and diversification of the Laverania subgenus. Proc Natl Acad Sci USA 107(23):10561–10566.OpenUrlAbstract/FREE Full Text↵Rayner JC, Liu W, Peeters M, Sharp PM, Hahn BH (2011) A plethora of Plasmodium species in wild apes: A source of human infection? Trends Parasitol 27(5):222–229.OpenUrlCrossRefPubMed↵Krief S, et al. (2010) On the diversity of malaria parasites in African apes and the origin of Plasmodium falciparum from Bonobos. PLoS Pathog 6(2):e1000765.OpenUrlCrossRefPubMed↵Liu W, et al. (2010) Origin of the human malaria parasite Plasmodium falciparum in gorillas. Nature 467(7314):420–425.OpenUrlCrossRefPubMed↵Ollomo B, et al. (2009) A new malaria agent in African hominids. PLoS Pathog 5(5):e1000446.OpenUrlCrossRefPubMed↵Prugnolle F, et al. (2011) Plasmodium falciparum is not as lonely as previously considered. Virulence 2(1):71–76.OpenUrlCrossRefPubMed↵Blacklock B, Adler S (1922) A parasite resembling Plasmdium falciparum in a chimpanzee. Ann Trop Med Parasitol 16:99–107.OpenUrl↵Daubersies P, et al. (2000) Protection against Plasmodium falciparum malaria in chimpanzees by immunization with the conserved pre-erythrocytic liver-stage antigen 3. Nat Med 6(11):1258–1263.OpenUrlCrossRefPubMed↵Martin MJ, Rayner JC, Gagneux P, Barnwell JW, Varki A (2005) Evolution of human-chimpanzee differences in malaria susceptibility: Relationship to human genetic loss of N-glycolylneuraminic acid. Proc Natl Acad Sci USA 102(36):12819–12824.OpenUrlAbstract/FREE Full Text↵Birkholtz LM, et al. (2008) Heterologous expression of plasmodial proteins for structural studies and functional annotation. Malar J 7:197.OpenUrlCrossRefPubMed↵Collins WE, Skinner JC, Pappaioanou M, Broderson JR, Mehaffey P (1986) The sporogonic cycle of Plasmodium reichenowi. J Parasitol 72(2):292–298.OpenUrlCrossRefPubMed↵Miller LH, Baruch DI, Marsh K, Doumbo OK (2002) The pathogenic basis of malaria. Nature 415(6872):673–679.OpenUrlCrossRefPubMed↵Cowman AF, Crabb BS (2006) Invasion of red blood cells by malaria parasites. Cell 124(4):755–766.OpenUrlCrossRefPubMed↵Jeffares DC, et al. (2007) Genome variation and evolution of the malaria parasite Plasmodium falciparum. Nat Genet 39(1):120–125.OpenUrlCrossRefPubMed↵Bannister L, Mitchell G (2003) The ins, outs and roundabouts of malaria. Trends Parasitol 19(5):209–213.OpenUrlCrossRefPubMed↵Bartholdson SJ, Crosnier C, Bustamante LY, Rayner JC, Wright GJ (2013) Identifying novel Plasmodium falciparum erythrocyte invasion receptors using systematic extracellular protein interaction screens. Cell Microbiol 15(8):1304–1312.OpenUrlCrossRefPubMed↵Harvey KL, Gilson PR, Crabb BS (2012) A model for the progression of receptor-ligand interactions during erythrocyte invasion by Plasmodium falciparum. Int J Parasitol 42(6):567–573.OpenUrlCrossRefPubMed↵Duraisingh MT, Maier AG, Triglia T, Cowman AF (2003) Erythrocyte-binding antigen 175 mediates invasion in Plasmodium falciparum utilizing sialic acid-dependent and -independent pathways. Proc Natl Acad Sci USA 100(8):4796–4801.OpenUrlAbstract/FREE Full Text↵Narum DL, et al. (2000) Antibodies against the Plasmodium falciparum receptor binding domain of EBA-175 block invasion pathways that do not involve sialic acids. Infect Immun 68(4):1964–1966.OpenUrlAbstract/FREE Full Text↵Muchmore EA, Diaz S, Varki A (1998) A structural difference between the cell surfaces of humans and the great apes. Am J Phys Anthropol 107(2):187–198.OpenUrlCrossRefPubMed↵Tolia NH, Enemark EJ, Sim BK, Joshua-Tor L (2005) Structural basis for the EBA-175 erythrocyte invasion pathway of the malaria parasite Plasmodium falciparum. Cell 122(2):183–193.OpenUrlCrossRefPubMed↵Chattopadhyay D, Rayner J, McHenry AM, Adams JH (2006) The structure of the Plasmodium falciparum EBA175 ligand domain and the molecular basis of host specificity. Trends Parasitol 22(4):143–145.OpenUrlCrossRefPubMed↵Orlandi PA, Klotz FW, Haynes JD (1992) A malaria invasion receptor, the 175-kilodalton erythrocyte binding antigen of Plasmodium falciparum recognizes the terminal Neu5Ac(alpha 2-3)Gal- sequences of glycophorin A. J Cell Biol 116(4):901–909.OpenUrlAbstract/FREE Full Text↵Wanaguru MK, Crosnier C, Johnson S, Rayner JC, Wright GJ (2013) A biochemical analysis of the Plasmodium falciparum Erythrocyte Binding Antigen-175 (EBA175) - Glycophorin-A interaction: Implications for vaccine design. J Biol Chem 288(45):32106–32117.↵Hayton K, et al. (2013) Various PfRH5 polymorphisms can support Plasmodium falciparum invasion into the erythrocytes of owl monkeys and rats. Mol Biochem Parasitol 187(2):103–110.OpenUrlCrossRefPubMed↵Hayton K, et al. (2008) Erythrocyte binding protein PfRH5 polymorphisms determine species-specific pathways of Plasmodium falciparum invasion. Cell Host Microbe 4(1):40–51.OpenUrlCrossRefPubMed↵Crosnier C, et al. (2011) Basigin is a receptor essential for erythrocyte invasion by Plasmodium falciparum. Nature 480(7378):534–537.OpenUrlPubMed↵Crosnier C, et al. (2013) A library of functional recombinant cell surface and secreted Plasmodium falciparum merozoite proteins. Mol Cell Proteomics, in press.↵Bartholdson SJ, et al. (2012) Semaphorin-7A is an erythrocyte receptor for P. falciparum merozoite-specific TRAP homolog, MTRAP. PLoS Pathog 8(11):e1003031.OpenUrlCrossRefPubMed↵Bushell KM, Söllner C, Schuster-Boeckler B, Bateman A, Wright GJ (2008) Large-scale screening for novel low-affinity extracellular protein interactions. Genome Res 18(4):622–630.OpenUrlAbstract/FREE Full Text↵Yu XL, et al. (2008) Crystal structure of HAb18G/CD147: Implications for immunoglobulin superfamily homophilic adhesion. J Biol Chem 283(26):18056–18065.OpenUrlAbstract/FREE Full Text↵Schlegel J, et al. (2009) Solution characterization of the extracellular region of CD147 and its interaction with its enzyme ligand cyclophilin A. J Mol Biol 391(3):518–535.OpenUrlCrossRefPubMed↵Sun Y, Gallagher-Jones M, Barker C, Wright GJ (2012) A benchmarked protein microarray-based platform for the identification of novel low-affinity extracellular protein interactions. Anal Biochem 424(1):45–53.OpenUrlCrossRefPubMed↵Sim BK, et al. (2011) Delineation of stage specific expression of Plasmodium falciparum EBA-175 by biologically functional region II monoclonal antibodies. PLoS ONE 6(4):e18393.OpenUrlCrossRefPubMed↵Koch C, et al. (1999) T cell activation-associated epitopes of CD147 in regulation of the T cell response, and their definition by antibody affinity and antigen density. Int Immunol 11(5):777–786.OpenUrlAbstract/FREE Full Text↵Kerr JS, Wright GJ (2012) Avidity-based extracellular interaction screening (AVEXIS) for the scalable detection of low-affinity extracellular receptor-ligand interactions. J Vis Exp 61:e3881.OpenUrlPubMed↵Wright GJ, et al. (2000) Lymphoid/neuronal cell surface OX2 glycoprotein recognizes a novel receptor on macrophages implicated in the control of their function. Immunity 13(2):233–242.OpenUrlCrossRefPubMed Thank you for your interest in spreading the word on PNAS.NOTE: We only request your email address so that the person you are recommending the page to knows that you wanted them to see it, and that it is not junk mail. We do not capture any email address.CAPTCHAThis question is for testing whether or not you are a human visitor and to prevent automated spam submissions. RH5–Basigin in Plasmodium host tropism Madushi Wanaguru, Weimin Liu, Beatrice H. Hahn, Julian C. Rayner, Gavin J. Wright Proceedings of the National Academy of Sciences Dec 2013, 110 (51) 20735-20740; DOI: 10.1073/pnas.1320771110 RH5–Basigin in Plasmodium host tropism Madushi Wanaguru, Weimin Liu, Beatrice H. Hahn, Julian C. Rayner, Gavin J. Wright Proceedings of the National Academy of Sciences Dec 2013, 110 (51) 20735-20740; DOI: 10.1073/pnas.1320771110 Sign up for the PNAS Highlights newsletter to get in-depth stories of science sent to your inbox twice a month: Relatively clean snow and ice in the Indus River Basin during the COVID-19 pandemic may have reduced meltwater in 2020, compared with the 20-year average. Atmospheric and climate conditions could have created a cloud greenhouse effect to warm Mars and support liquid surface water. Researchers report a safety guideline to limit airborne transmission of COVID-19 that goes beyond the six-foot social distancing guideline. Interventions include using rice husks, manipulating paddy water and soil, and genetic changes that could stop arsenic from reaching the grain. Going beyond conventional approaches, researchers are using carefully cultured bacterial communities to improve sewage treatment.
本文链接: https://www.ebiomall.cn/b526-immunetech/info-53662.html
免责声明 本文仅代表作者个人观点,与本网无关。其创作性以及文中陈述文字和内容未经本站证实,对本文以及其中全部或者部分内容、文字的真实性、完整性、及时性本站不做任何保证或承诺,请读者仅作参考,并请自行核实相关内容。
版权声明 未经蚂蚁淘授权不得转载、摘编或利用其他方式使用上述作品。已经经本网授权使用作品的,应该授权范围内使用,并注明“来源:蚂蚁淘”。违反上述声明者,本网将追究其相关法律责任。
相关文章
1970-01-01
1970-01-01
1970-01-02
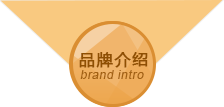
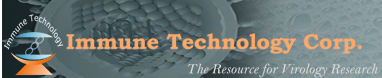
▍
品牌分类
▍
官网分类
▍
品牌问答